FELINE INFECTIOUS PERITONITIS
To My Quyen, Tran Duy Thanh, Pham Trang Thanh Nguyen,
Nguyen Khanh Thuan, Nguyen Phuc Khanh, Nguyen Thanh Lam*
1. Introduction
Feline infectious peritonitis (FIP) is a progressive systemic disease characterized by a wide range of clinical symptoms, represented by peritonitis, the accumulation of a large amount of ascites, and a high mortality rate (Pedersen et al., 1981). It is highly contagious and spreads efficiently through fecal–oral transmission, leading to a high prevalence in multi-cat environments such as breeding catteries (Hayashi et al., 1982), shelter or rescue facilities, and situations involving animal hoarding (Addie and Jarrett, 1992). FIP, a viral-induced immune-mediated disease with a high fatality rate, is caused by the feline infectious peritonitis virus (FIPV). FIPV is a member of the genus Alphacoronavirus, a group of enveloped, single-stranded, positive-sense RNA viruses, stemming from a mutation in the feline coronavirus (FCoV) (Addie and Jarrett, 2001; Kipar et al., 1998). FIP was first identified as a distinct disease in 1963 by Dr. Jean Holzworth et al. at the Angell Memorial Animal Hospital in Boston, USA. Feline coronavirus (FCoV) is prevalent worldwide and is commonly found in most cat populations (Kipar et al., 2010).
2. Aetiology
2.1 Classification
Feline coronavirus (FCoV) is a large, pleomorphic spherical, enveloped virus particle classified in the order Nidovirales; family Coronaviridae; subfamily Coronavirinae; genus Alphacoronavirus; species Alphacoronavirus 1, which also includes the enteritis-causing canine coronavirus (CCoV), transmissible gastroenteritis virus (TGEV) and porcine respiratory coronavirus (PRCoV) (de Groot et al., 2011; Jaimes et al., 2020). The newly emerged severe acute respiratory syndrome coronavirus 2 (SARS-CoV-2) is very distinct and different from FCoV, belonging to a different genus: the genus Betacoronavirus(Haake et al., 2020). Members of the Coronaviridae family are grouped in four genera, where Alphacoronavirus and Betacoronavirus include viruses that principally infect mammals, and are derived from the bat gene pool; whereas Gammacoronavirus and Deltacoronavirus group viruses that infect birds and mammals and are derived from the avian and pig gene pool (Table 1) (Jaimes and Whittaker, 2018).
Table 1: Genus and species from Coronavirinae subfamily.
Genus | Species | Viruses |
Alphacoronavirus | Alphacoronavirus 1 | Transmissible gastroenteritis virus (TEGV) |
|
| Feline coronavirus (FCoV) |
| Porcine epidemic diarrhea virus (PEDV) | |
| Human coronavirus NL63 (HCoV-NL63) | |
| Human coronavirus 229E (HCoV-229E) | |
Betacoronavirus | Betacoronavirus 1 | Bovine coronavirus (BCoV) |
|
| Human coronavirus OC43 (HCoV-OC43) |
| Middle East respiratory syndrome-related coronavirus (MERS-CoV) | |
| Murine coronavirus | Mouse hepatitis virus (MHV) |
| Severe acute respiratory syndrome-related coronavirus (SARS-CoV) | |
| Human coronavirus HKU1 (HCoV-HKU1) | |
Gammacoronavirus | Avian coronavirus | Infectious bronchitis virus (IBV) |
|
| Turkey coronavirus |
| Beluga whale coronavirus SW1 | |
Deltacoronavirus | Coronavirus HKU15 (also known as porcine coronavirus PCoV-HKU15) |
2.2 Viral characteristics
Being an enveloped virus, FCoV is readily inactivated by most disinfectants, steam, and washing at 60°C (Addie et al., 2015a). It has been suggested it might preserve its infectivity for days to a few weeks (Scott, 1988), depending on environmental conditions and protection by faecal matter. A schematic diagram of the FCoV genome is shown in Figure 1.
Figure 1: Schematic diagrams of type I FcoV (Tasker et al., 2023).
(a) Schematic FCoV genome. FCoV is a positive-sense single-stranded RNA virus. The FCoV genome of 27–32 kilobases encodes a replicase polyprotein, four structural proteins (spike [S], membrane [M], nucleocapsid [N] and envelope [E]) and non-structural accessory proteins 3a, 3b and 3c and 7a and 7b. UTR indicates an untranslated region. Image Emi Barker, Langford Vets, University of Bristol, UK. (b) Schematic FCoV spike protein sequence showing the division into the S1 and S2 subunits representing the receptor-binding and fusion domains, respectively, with N- and C-terminals shown. The S1/S2 and S2′ sites represent cleavage sites (in red), and the fusion peptide domain is also shaded in red. The positions of the M1058* and S1060* amino acid residues (blue lines) are shown because these correspond to the FCoV nucleotide sequences in specific spike gene mutations that are evaluated in some commercially available molecular assays. * Convention is to label amino acid substitutions by initials surrounding the numbered amino acid residue location (e.g., M1058L indicates that methionine is replaced by leucine at position 1058; similarly, S1060A indicates that serine is replaced by alanine at position 1060). Image Séverine Tasker, University of Bristol, UK.
The 5′ two-thirds of the positive-sense coronavirus genome consist of two overlapping open reading frames (ORFs), 1a and 1b, that encode non-structural polyprotein (pp) 1 (pp1a and pp1b) (Figure 1a). The polyproteins are cleaved into individual non-structural proteins (nsps), including RNA-dependent RNA polymerase that plays a role in viral replication. ORF 1a also encodes for viral proteases, including the viral 3C-like protease, which is a target for antiviral therapy. The other third of the genome consists of ORFs encoding structural proteins, a spike [S] (a protein found on the FCoV surface – Figure 1b), a membrane (in the FCoV membrane), a nucleocapsid (the protein wrapped around the FCoV genome), an envelope (also in the FCoV membrane) (Figure 2) and non-structural accessory proteins 3a, 3b, 3c, 7a and 7b (Figure 1a) (Horzinek and Lutz, 2001; Terada et al., 2014). Non-structural proteins are involved in the replication of the virus and modification of the host immune response but are not incorporated into the mature virus particle (Tasker et al., 2023).
Figure 2: Schematic diagram of FCoV structure showing single-stranded RNA and the structural proteins: spike, envelope, membrane and nucleocapsid proteins. The spike protein is the part of the virus particle that interacts with the host-cell receptor. The spikes on the surface present a coronal (i.e., crown-like) appearance under electron microscopy (Jaimes and Whittaker, 2018).
3. Epidemiology
FCoV is a contagious virus. Faeces are the main source of FCoV infection and most transmission is faecal-oral in nature.
Kittens are often infected at a young age and shed FCoV in faeces as early as two days post-infection. After infection, shedding continues for days, weeks or months, and a few may be persistently infected. Shedding then stops, or is detected intermittently, and can recur due to re-infection in an endemic environment. Immunity is short-lived, which is why cats, in the face of infection, can undergo multiple cycles of infections.
FCoV infection occurs worldwide (Table 2) and is very common, particularly in multi-cat households, but FIP arises in only a small percentage of FCoV-infected cats. Cats of any breed or age can develop FIP. It is particularly seen in pedigree cats (especially in certain breeds in some studies) and those under 2 years of age. In some studies, males were more likely to develop FIP than females (Tasker et al., 2023).
Table 2: Prevalence of FCoV in various countries from selected studies using either serum FCoV antibody or faecal FCoV RNA detection.
Country | Method used for prevalence determination | Number of Cats | Prevalence | Year of study |
Australia | Antibodies | 49 feral cats 306 owned cats | 0% 34% | 2006 |
Australia | FCoV RNA in faeces | 289 cats with diarrhea including: 80 shelter cats with diarrhea | 40% 54% | 2019 |
Croatia | Antibodies | 106 pet cats | 42% | 2021 |
Czech Republic | FCoV RNA in faeces | 70 shelter cats | 63% | 2022 |
Falkland Islands | Antibodies | 10 feral cats 95 pet cats | 0% 0% | 2012 |
France | FCoV RNA in faeces | 88 healthy cats | 17% | 2013 |
Galapagos Islands | Antibodies | 34 pet and 18 feral cats | 0% | 2008 |
Germany | FCoV RNA in faeces | 82 cats from 19 breeding catteries | 71% | 2020 |
Germany | Antibodies | 82 cats from 19 breeding catteries | 78% | 2020 |
3.2 Transmissions
FCoV is shed mainly in the feces. In early infection, it may be found in saliva when the virus replicates in tonsils and, possibly, in respiratory secretions and urine (Herrewegh et al., 1995; Stoddart et al., 1988). It is likely that when naive cats in a multiple-cat household first encounter FCoV all become infected (and develop antibodies) and most probably shed virus for a period of weeks or months. With extremely sensitive reverse transcriptase (RT)–polymerase chain reaction (PCR) techniques, it has been shown that many naturally infected healthy carrier cats shed FCoV for at least up to 10 months (Herrewegh et al., 1995). Most cats shed virus intermittently, but some become chronic FCoV shedders for years to lifelong, providing a continuous source for reinfection of other cats. Cats that are antibody-negative are unlikely to shed, whereas approximately one third of FCoV antibody–positive cats shed virus. It has been shown that cats with high antibody titers are more likely to shed FCoV and to shed more consistently and higher amounts of the virus (Lutz et al., 2002). Thus, the height of the titer is directly correlated with virus replication and the amount of virus in the intestines. Most cats with FIP also shed (nonmutated) FCoV; however, the virus load in feces seems to decrease after a cat has developed FIP (Lutz et al., 2002).
4. Pathogenesis
It has been reported that the occurrence of effusive or non-effusive form depends on immune status (Paltrinieri et al., 1998). Studies have shown that FIPV infections caused by both inoculation in the laboratory and natural infection have very short effusive FIP periods. Observations of experimentally and naturally infected cats have shown that lesions associated with non-effusive FIP are remnants of lesions associated with effusive FIP, which indicates that during the initial stage of FIPV infection, a short episode of effusive FIP might develop first because of the weak immune response to the virus, while with the improvement of the immune response, effusive FIP should gradually transform to non-effusive FIP (Pedersen, 2009). When the immune system is suppressed, non-effusive FIP can transform into effusive FIP. In the final stage of the disease, the occurrence of non-effusive FIP is presumably due to partial protection by the immune system. Whether effusive or non-effusive FIP occurs may be related to whether the body initiates a cellular or humoral immune response in the early stage of infection. Clinical disease symptoms do not occur if cellular immunity is activated early and if the immune response is sufficient, although viral replication can still be detected. At this time, the infection often progresses to non-effusive FIP. If a humoral immune response occurs and cellular immune initiation fails, the final form of the disease is often effusive or wet FIP. Nevertheless, in many cases, the host mounts an immune response in the middle stage of infection, initiating strong humoral immunity and weak cellular immunity (Pedersen, 2009). At this time, both virus replication and virus eradication occur. As a result, non-effusive FIP develops, and typical granulomas form. In the centers of the granulomas are small aggregations of macrophages that generally do not contain virus antigens or contain only small amounts of virus antigens surrounded by a broad band of lymphocytes or plasma cells. Effusive FIP can lead to the formation of pyogranulomas covered with virus-loaded macrophages and scattered neutrophils and lymphocytes. Pyogranulomas extend from the surface of an organ into the underlying parenchyma. The distribution of dry FIP granulomas in organs is related to a partial or weak immune status. At the initial stage of FIPV invasion, the virus reaches the brain and eyes through infected monocytes. However, because of the blood‒brain barrier, immune cells have difficulty reaching the brain. If protective immunity is strong enough, virus particles or virus-infected cells are quickly eradicated; if immunity is weak, immune cells are much more effective in dealing with virus particles and virus-infected cells in the core of the body than they will be in the brain or eyes due to the blood‒brain barrier. Thus, lesions in the brain and eyes last longer than those in the abdomen or other parts of the body, which explains why dry FIP is more likely than wet FIP to occur in the brain and eyes (Figure 3).
Figure 3: Schematic representation of the pathogenesis of effusive and non-effusive FIP (Pedersen, 2009).
5. Clinical signs and pathology
5.1 Clinical signs
FHV-1 causes upper respiratory tract disease (URTD) and conjunctivitis or keratitis via its cytopathic effect on epithelial cells. Cats affected by both primary infection and viral recrudescence are likely to have ocular disease. Conjunctivitis is the most common ocular condition (Figure 4), followed by corneal epithelial ulceration and keratitis, with or without ulceration (Figure 5). Conjunctivitis is manifested by conjunctival hyperemia, with or without chemosis, tearing and discomfort. The nictitating membrane can sometimes be elevated due to pain or swelling. The early inflammatory response is neutrophilic and a purulent ocular discharge is common. Dendritic epithelial corneal ulceration is the classic herpetic lesion and, if evident, is helpful in making the diagnosis. However, many cats with FHV-1-related ocular disease are presented with geographic epithelial ulcers. Both of these types of epithelial ulcers can be seen after staining with fluorescein. Rose Bengal will highlight dendritic ulcers, but is irritating and a topical anesthetic should be used before this stain is applied. Keratitis without corneal ulceration can also occur in cats, manifesting as corneal vascularization, with or without inflammatory cell infiltrates (Figure 5). Corneal disease from FHV-1 is almost always accompanied by conjunctivitis. Some cats with FCV infection have severe conjunctivitis that often precludes visualization of the cornea (Figure 6). Infection with FIV is associated with lymphosarcoma in cats (Gabor et al, 2001, Magden et al, 2013), with the potential for ocular involvement (Figure 7). Ocular lymphosarcoma can involve the uveal tract, conjunctiva or orbit (Stiles, 2014).
Other corneal conditions, such as sequestra and eosinophilic keratitis, have been associated with FHV-1 (Nasisse et al, 1998, Dean, Meunier, 2013). These conditions are more likely to occur with chronic FHV-1-associated ocular disease. Typically, primary infection with FHV-1 is self-limiting, lasting a few weeks, followed by clinical recovery. However, some cats develop chronic conjunctivitis that does not resolve spontaneously. Chronic conjunctivitis, or recurrent conjunctivitis, can occur bilaterally, but it is not uncommon for only one eye to be affected. This might lead the clinician to erroneously assume that FHV-1 could not be the underlying pathogen.
Figure 4: Conjunctivitis caused by feline herpesvirus type 1 in an adult cat. Note conjunctival hyperemia, chemosis and purulent ocular discharge. The diagnosis was made based on virus isolation from a conjunctival swab (Stiles, 2014).
Figure 5: Keratitis without corneal ulceration caused by feline herpesvirus type 1 in a kitten. Note corneal vascularization and edema. Conjunctivitis is also present. The diagnosis was made based on virus isolation and positive PCR results from a conjunctival swab (Stiles, 2014).
Figure 6: Severe conjunctivitis caused by feline calicivirus in a kitten. Note marked conjunctival hyperemia and chemosis that obscures the cornea. The diagnosis was made based on virus isolation from a conjunctival swab (Stiles, 2014).
Figure 7: Uveal lymphosarcoma in a cat positive for feline immunodeficiency virus (FIV). Note the cellular infiltrate in the iris and iridal vascular congestion. The diagnosis was made based on finding neoplastic lymphocytes on an aqueous humor sample and a positive serum antibody test for FIV (Stiles, 2014).
5.2 Pathology
Grossly, the wet/effusive form of FIP is characterized by “straw-colored,” semi-translucent, protein-rich peritoneal or thoracic effusions and fibrinous and granulomatous serositis/pleuritis with variable involvement of parenchymal organs (Figure 8). Virus-associated pyogranulomatous inflammation is focused on small and medium sized veins, resulting in vascular injury and leakage (Kipar et al., 2005). These vasculo-centric lesions can occur in the omentum and serosal surfaces of the liver, spleen, intestines, kidneys, and lungs, and are composed primarily of macrophage aggregates in combination with smaller numbers of neutrophils and lymphocytes (pyogranulomatous inflammation). Occasionally, pyogranulomatous nodular lesions extend beyond the serosal surfaces into underlyingparenchyma. Corona viral antigen can often be detected within intralesional macrophages using immunohistochemistry techniques; corona viral antigen detection via immunohistochemistry is a commonly utilized diagnostic method.
The dry form of FIP is characterized grossly by variably sized parenchymal and serosal pyogranulomas in affected organs but lacks the exudation archetypal of wet FIP. Granulomatous lesions of dry FIP may extend from serosal surfaces into the parenchyma of affected organs, and lesions may be restricted to a single organ, such as the kidney, eye, or brain. Other frequently affected organs in the dry form of FIP include the mesenteric and mediastinal lymph nodes, omentum, intestine, and liver. Perivascular inflammatory lesions contain aggregates of macrophages and fewer neutrophils, which are surrounded by dense infiltrates of primarily B lymphocytes and plasma cells extending into surrounding tissues, with or without the presence of vasculitis (Drechsler et al., 2011). It is not uncommon for affected animals to demonstrate some combination of both effusive and granulomatous forms of the disease.
Figure 8: (A) Gross image of “wet” or effusive feline infectious peritonitis, thoracic and abdominal cavities, cat. Abundant semi-translucent “straw-colored”, proteinaceous peritoneal effusion with fibrinous and granulomatous serositis and multifocal granulomatous lesions in the liver. Gross image courtesy of Chrissy Eckstrand. (B) FIP, urinary bladder serosal surface, cat, hematoxylin and eosin (HE). Severe, necrotizing, pyogranulomatous and lymphoplasmacytic serositis and vasculitis. (C) FIP, urinary bladder serosal surface, cat, FCoV immunohistochemistry. Same lesion tissue as 3b with frequent, positive immune reactivity for FCoV antigen (brown pigment).
6. Diagnosis
6.1 Clinical symptoms
In cases of effusive FIP, abdominal swelling can be seen. An obvious fluid wave can be felt upon palpation, but it is necessary to consider the differential diagnosis of pregnancy in cats or other circumstances that cause abdominal swelling. Sometimes uveitis and neurological symptoms occur, but an exact diagnosis cannot be made according to only these symptoms. For noneffusive FIP, although lesions in the eye can occur, they are not characteristic. The diagnosis of FIP requires histological, viral antigen, physiological, biochemical, serological and molecular biological examinations after the death of the sick cat (Giori et al., 2011; Thayer et al., 2022).
6.2 Serologic detection
FcoV infection causes antibody production independent of the development of FIP. For FCoV infection established under laboratory conditions, serum antibodies can be detected from 7 to 28 days after infection (Felten et al., 2019; Vogel et al., 2010). Anti-FCoV antibodies were first detected in 1976. Since then, many methods have been established for antibody detection, including indirect fluorescent antibody tests (IFATs) using FCoV or porcine infectious gastroenteritis virus (TGEV) as the antigen, virus neutralization (VN), enzyme-linked immunosorbent assay (ELISA), and rapid immunochromatography (RIM) (Addie et al., 2015b). Ideally, tests should be performed on different fluids, such as serum and effusion fluid, and high specificity and sensitivity are needed.
6.3 Immunohistochemical method
Immunohistochemistry (IHC) is a method used to detect antigens via immunological and biochemical staining of tissues with characteristic histopathological changes caused by FIP. This method has been used to detect lesions in cats with FIP for more than 20 years, and the sensitivity of this detection method is 97–100%. Therefore, this method is considered to be the gold standard for the diagnosis of FIP, and it can be used to suggest the presence of viruses in tissues with histopathological lesions and to diagnose FIP in atypical cases (Drechsler et al., 2011; Felten et al., 2019).
7. Prevention
There has been a delay in FIPV vaccine development because of ADE, which causes vaccinated animals to produce antibodies that actually benefit virus infection. There is only one FCoV vaccine available; it requires nasal inoculation and is used mainly in the United States and some European countries. The vaccine contains a temperature-sensitive FCoV II mutant strain, DF2-FIPV. However, since FCoV I is more prevalent than FCoV II, the vaccine's protective effect remains controversial. Takano and coworkers (Takano et al., 2014) recently designed a polypeptide vaccine consisting of T helper epitopes derived from the nuclear protein of FIPV KU-2; this vaccine overcomes the drawbacks of ADE. However, the effectiveness of the vaccine still needs to be improved. Routine precautions against FIP include blockade of its route of transmission. If cats with FIP die in a house, it is recommended that the owner thoroughly disinfects the environment and does not obtain a new cat for at least two months so that all the FIPV particles in the environment die or become inactivated. In addition, to ensure a healthy living environment for cats, the dwelling should be kept clean and disinfected regularly, overcrowding should be avoided (no more than 3 cats per room), and stress should be prevented in cats. In addition, care should be taken when cats from different environments mingle, especially when a new cat is introduced, and any FCoV infections should be identified in existing and new cats. Only through routine cleaning can the source of FIP be eliminated (Foley et al., 1997).
8. Treatment
There are no specific drugs to treat FIP, and symptomatic treatment can only relieve symptoms and prolong life. Tylosin and prednisolone were the first reported drugs to be used to cure cats with FIP. However, tylosin has no effect on FIP after a long decade of treatment (Colgrove and Parker, 1971; Pedersen, 2009). It has been reported that some cats have gone into remission after the use of prednisolone and phenylalanine mustard or cyclophosphamide with anti-inflammatory and anti-allergic effects, and injections of antibiotics such as ampicillin and ceftiofur can prevent secondary bacterial infection (Pedersen, 1976a). FIPV is reportedly sensitive to human α- and β-interferon in vitro (Weiss and Toivio-Kinnucan, 1988). In addition, ω-interferon also inhibits FIPV in vitro, and the drug has been used in some countries (Mochizuki et al., 1994). However, a large double-blind experiment indicated that the drug is generally ineffective (Ritz et al., 2007).
8.1 Antiviral treatment
The nucleoside analogue GS-441524 is the active metabolite of the prodrug remdesivir (GS-5734). Both remdesivir and GS-441524 have been found to be effective for FIP treatment (Taylor et al., 2023). Reported dosages are 2–15 mg/kg, IM, every 24 hours for at least 12 weeks.
These drugs hamper viral replication via delayed chain termination. Once the drug is inside the cell, phosphorylation by cellular kinases produces a nucleoside monophosphate and then an active nucleoside triphosphate metabolite, which competes with natural nucleoside triphosphates used as substrates in viral RNA synthesis. Incorporation of the nucleoside analogue into the nascent viral RNA transcript results in the premature termination of the nucleic acid chain.
GS-441524 remains under patent and is not approved for veterinary use in some countries. Unlicensed GS-441524–like antiviral drugs for oral administration are commercially available. Reported adverse effects include injection-site discomfort, nonprogressive renal toxicosis, steroid-responsive vasculitis-type reactions, and urolithiasis (Murphy et al., 2018; Pedersen et al., 2019).
Clinical improvement of FIP has been demonstrated with the 3c-like protease inhibitor GC376 (15 mg/kg, SC, every 12 hours for 12 weeks). In the US, FDA approval is pending. Adverse effects of GC376 include injection-site discomfort and interference with adult dentition development in kittens (Kim et al., 2016; Kim et al., 2015).
Clinical remission of FIP has been reported with the nucleoside analogue molnupiravir (12.8–14.6 mg/kg, PO, every 12 hours for 10–15 weeks [median, 12 weeks]) as a rescue treatment in cats with persistent or relapsed clinical signs of FIP after treatment with GS-441524 or GC376. Reported adverse effects of molnupiravir include folded ears, broken whiskers, and leukopenia (Roy et al., 2022; Sase, 2023)
FCoV replication is inhibited by feline interferon omega in vitro; however, no effect on survival time or quality of life has been demonstrated. Additional study is needed to determine efficacy in vivo (Ritz et al., 2007).
Other drugs that inhibit viral replication, including ribavirin, vidarabine, and nelfinavir, are not recommended, because they are toxic in cats.
8.2 Supportive care
Treatment of FIP with corticosteroids (prednisolone at 2–4 mg/kg, PO, every 24 hours) or cyclophosphamide (2–4 mg/kg, PO, every 48 hours), alone or in combination, may result in temporary clinical improvement or slowed progression of the disease. Use of chlorambucil has also been reported.
Thoracic effusions are usually associated with dyspnea and should be drained via thoracocentesis. Other than for sampling, the volume of abdominal effusion should be drained via abdominocentesis only if it is interfering with respiration; drained effusion fluid will rapidly reaccumulate.
Nonspecific supportive care for FIP includes fluid therapy, nutritional support, and ancillary treatments such as administration of vitamin B12. Ocular disease and other concurrent illness should be treated. Antimicrobials are indicated only for secondary bacterial infections (Roman, 2024).
9. References
Addie, D., Jarrett, O., 1992. A study of naturally occurring feline coronavirus infections in kittens. The Veterinary Record 130, 133-137.
Addie, D., Jarrett, O., 2001. Use of a reverse‐transcriptase polymerase chain reaction for monitoring the shedding of feline coronavirus by healthy cats. Veterinary Record 148, 649-653.
Addie, D.D., Boucraut-Baralon, C., Egberink, H., Frymus, T., Gruffydd-Jones, T., Hartmann, K., Horzinek, M.C., Hosie, M.J., Lloret, A., Lutz, H., 2015a. Disinfectant choices in veterinary practices, shelters and households: ABCD guidelines on safe and effective disinfection for feline environments. Journal of feline medicine and surgery 17, 594-605.
Addie, D.D., Le Poder, S., Burr, P., Decaro, N., Graham, E., Hofmann-Lehmann, R., Jarrett, O., McDonald, M., Meli, M.L., 2015b. Utility of feline coronavirus antibody tests. Journal of feline medicine and surgery 17, 152-162.
de Groot, R.J., Baker, S., Baric, R., Enjuanes, L., Gorbalenya, A., Holmes, K., Perlman, S., Poon, L., Rottier, P., Talbot, P., 2011. Family coronaviridae.
Drechsler, Y., Alcaraz, A., Bossong, F.J., Collisson, E.W., Diniz, P.P.V., 2011. Feline coronavirus in multicat environments. Veterinary Clinics: Small Animal Practice 41, 1133-1169.
Felten, S., Hartmann, K., Doerfelt, S., Sangl, L., Hirschberger, J., Matiasek, K., 2019. Immunocytochemistry of mesenteric lymph node fine-needle aspirates in the diagnosis of feline infectious peritonitis. Journal of Veterinary Diagnostic Investigation 31, 210-216.
Foley, J.E., Poland, A., Carlson, J., Pedersen, N.C., 1997. Risk factors for feline infectious peritonitis among cats in multiple-cat environments with endemic feline enteric coronavirus. Journal of the American Veterinary Medical Association 210, 1313-1318.
Giori, L., Giordano, A., Giudice, C., Grieco, V., Paltrinieri, S., 2011. Performances of different diagnostic tests for feline infectious peritonitis in challenging clinical cases. Journal of Small Animal Practice 52, 152-157.
Haake, C., Cook, S., Pusterla, N., Murphy, B., 2020. Coronavirus infections in companion animals: virology, epidemiology, clinical and pathologic features. Viruses 12, 1023.
Hayashi, T., Watabe, Y., Nakayama, H., Fujiwara, K., 1982. Enteritis due to feline infectious peritonitis virus.
Herrewegh, A., De Groot, R., Cepica, A., Egberink, H.F., Horzinek, M.C., Rottier, P., 1995. Detection of feline coronavirus RNA in feces, tissues, and body fluids of naturally infected cats by reverse transcriptase PCR. Journal of clinical microbiology 33, 684-689.
Horzinek, M.C., Lutz, H., 2001. An update on feline infectious peritonitis. Veterinary Sciences Tomorrow 1.
Jaimes, J.A., Millet, J.K., Stout, A.E., André, N.M., Whittaker, G.R., 2020. A tale of two viruses: the distinct spike glycoproteins of feline coronaviruses. Viruses 12, 83.
Jaimes, J.A., Whittaker, G.R., 2018. Feline coronavirus: Insights into viral pathogenesis based on the spike protein structure and function. Virology 517, 108-121.
Kim, Y., Liu, H., Galasiti Kankanamalage, A.C., Weerasekara, S., Hua, D.H., Groutas, W.C., Chang, K.-O., Pedersen, N.C., 2016. Reversal of the progression of fatal coronavirus infection in cats by a broad-spectrum coronavirus protease inhibitor. PLoS pathogens 12, e1005531.
Kim, Y., Shivanna, V., Narayanan, S., Prior, A.M., Weerasekara, S., Hua, D.H., Kankanamalage, A.C.G., Groutas, W.C., Chang, K.-O., 2015. Broad-spectrum inhibitors against 3C-like proteases of feline coronaviruses and feline caliciviruses. Journal of virology 89, 4942-4950.
Kipar, A., Kremendahl, J., Addie, D., Leukert, W., Grant, C., Reinacher, M., 1998. Fatal enteritis associated with coronavirus infection in cats. Journal of Comparative Pathology 119, 1-14.
Kipar, A., May, H., Menger, S., Weber, M., Leukert, W., Reinacher, M., 2005. Morphologic features and development of granulomatous vasculitis in feline infectious peritonitis. Veterinary pathology 42, 321-330.
Kipar, A., Meli, M.L., Baptiste, K.E., Bowker, L.J., Lutz, H., 2010. Sites of feline coronavirus persistence in healthy cats. Journal of general virology 91, 1698-1707.
Lutz, H., Gut, M., Leutenegger, C., Schiller, I., Wiseman, A., Meli, M., 2002. Kinetics of FCoV infection in kittens born in catteries of high risk for FIP under different rearing conditions, Second International Feline Coronavirus/Feline Infectious Peritonitis Symposium. Glasgow, Scotland.
Murphy, B., Perron, M., Murakami, E., Bauer, K., Park, Y., Eckstrand, C., Liepnieks, M., Pedersen, N.C., 2018. The nucleoside analog GS-441524 strongly inhibits feline infectious peritonitis (FIP) virus in tissue culture and experimental cat infection studies. Veterinary microbiology 219, 226-233.
Paltrinieri, S., Cammarata, M.P., Cammarata, G., Comazzi, S., 1998. Some aspects of humoral and cellular immunity in naturally occuring feline infectious peritonitis. Veterinary immunology and immunopathology 65, 205-220.
Pedersen, N.C., 2009. A review of feline infectious peritonitis virus infection: 1963–2008. Journal of feline medicine and surgery 11, 225-258.
Pedersen, N.C., Boyle, J., Floyd, K., Fudge, A., Barker, J., 1981. An enteric coronavirus infection of cats and its relationship to feline infectious peritonitis. American Journal of Veterinary Research 42, 368-377.
Pedersen, N.C., Perron, M., Bannasch, M., Montgomery, E., Murakami, E., Liepnieks, M., Liu, H., 2019. Efficacy and safety of the nucleoside analog GS-441524 for treatment of cats with naturally occurring feline infectious peritonitis. Journal of feline medicine and surgery 21, 271-281.
Ritz, S., Egberink, H., Hartmann, K., 2007. Effect of feline interferon‐omega on the survival time and quality of life of cats with feline infectious peritonitis. Journal of veterinary internal medicine 21, 1193-1197.
Roman, N., 2024. Feline Infectious Peritonitis. MSD Manual. Veterinary Manual.
Roy, M., Jacque, N., Novicoff, W., Li, E., Negash, R., Evans, S.J., 2022. Unlicensed molnupiravir is an effective rescue treatment following failure of unlicensed GS-441524-like therapy for cats with suspected feline infectious peritonitis. Pathogens (Basel, Switzerland) 11, 1209.
Sase, O., 2023. Molnupiravir treatment of 18 cats with feline infectious peritonitis: A case series. Journal of Veterinary Internal Medicine 37, 1876-1880.
Scott, F.W., 1988. Update on FIP, Proc. Kal. Kan. Symp, pp. 43-47.
Stiles, J., 2014. Ocular manifestations of feline viral diseases. The Veterinary Journal 201, 166-173.
Stoddart, M., Whicher, J., Harbour, D., 1988. Cats inoculated with feline infectious peritonitis virus exhibit a biphasic acute phase plasma protein response.
Takano, T., Tomizawa, K., Morioka, H., Doki, T., Hohdatsu, T., 2014. Evaluation of protective efficacy of the synthetic peptide vaccine containing the T-helper 1 epitope with CpG oligodeoxynucleotide against feline infectious peritonitis virus infection in cats. Antiviral Therapy 19, 645-650.
Tasker, S., Addie, D.D., Egberink, H., Hofmann-Lehmann, R., Hosie, M.J., Truyen, U., Belák, S., Boucraut-Baralon, C., Frymus, T., Lloret, A., 2023. Feline infectious peritonitis: European advisory board on cat diseases guidelines. Viruses 15, 1847.
Taylor, S.S., Coggins, S., Barker, E.N., Gunn-Moore, D., Jeevaratnam, K., Norris, J.M., Hughes, D., Stacey, E., MacFarlane, L., O’Brien, C., 2023. Retrospective study and outcome of 307 cats with feline infectious peritonitis treated with legally sourced veterinary compounded preparations of remdesivir and GS-441524 (2020–2022). Journal of feline medicine and surgery 25, 1098612X231194460.
Terada, Y., Matsui, N., Noguchi, K., Kuwata, R., Shimoda, H., Soma, T., Mochizuki, M., Maeda, K., 2014. Emergence of pathogenic coronaviruses in cats by homologous recombination between feline and canine coronaviruses. PloS one 9, e106534.
Thayer, V., Gogolski, S., Felten, S., Hartmann, K., Kennedy, M., Olah, G.A., 2022. 2022 AAFP/EveryCat feline infectious peritonitis diagnosis guidelines. Journal of Feline Medicine and Surgery 24, 905-933.
Vogel, L., Van der Lubben, M., Te Lintelo, E.G., Bekker, C.P., Geerts, T., Schuijff, L.S., Grinwis, G.C., Egberink, H.F., Rottier, P.J., 2010. Pathogenic characteristics of persistent feline enteric coronavirus infection in cats. Veterinary Research 41.
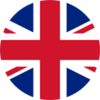