1. Introduction
Glasser disease is caused by infection with Glaesserella (Haemophilus) parasuis. The most common form is characterized by fibrinous polyserositis and polyarthritis, but septicemia with sudden death and bronchopneumonia also can occur. Diagnosis is based on clinical signs and bacterial isolation or PCR. Antibiotic treatment remains the first option to control a disease outbreak, but several vaccines are available for prevention (Segalés, 2020).
2. Aetiology
2.1 Viral characteristics
The etiological agent of Glässer’s disease was formally identified in 1931 as Haemophilus influenzae (variety suis), due to the similarity to the human pathogen H. influenzae. Later, Biberstein and White (1969) determined that contrary to H. influenzae, the swine bacterium did not require the X factor (hemin) to grow. Following the standard nomenclature in this group of microorganisms, H. influenzae (variety suis) was renamed H. parasuis. Haemophilus parasuis is a gram‐negative bacterium and member of the family Pasteurellaceae. Revision of the classification of some members of this family is ongoing, and reclassification of H. parasuis as a different genus has been proposed. In addition, high diversity in 16S rRNA gene sequence within the H. parasuis species and segregation into two distinct clusters have been reported (Angen et al., 2007).
2.2 Classification
H. parasuis strains are heterogeneous in phenotypic and genotypic traits, including virulence. Strains are classified into 15 serovars, although non‐typable isolates represent a high percentage. Serotyping can be performed by agar‐gel precipitation test (AGPT) or by indirect hemagglutination. H. parasuis serovars 4 and 5, along with non‐typable isolates, are reported as the most prevalent in different countries. Discrepancies in serotyping results are reported and are probably due to differences in methods, antisera, and reacting antigens. Recently, the analysis of loci encoding capsular polysaccharides from the 15 serovar reference strains has allowed design of polymerase chain reactions (PCRs) for molecular serotyping (Howell et al., 2013). These PCRs represent an invaluable tool for implementation of serotyping in many laboratories while reducing the number of non‐typable isolates and the inconsistency of results.
Several studies have attempted to correlate serovar and virulence, but a strict relationship between these two traits has not been demonstrated (Table 1). As an example, serovar 7 strains were thought to all be non‐virulent, but some serovar 7 strains have been isolated from systemic lesions of Glässer’s disease, and disease has been reproduced with one of them (Aragon et al., 2010).
In order to increase the discrimination capacity of the typing methods, several genotyping methods have been used to classify H. parasuis strains. H. parasuis was first genotyped using fingerprinting methods, such as restriction endonuclease pattern, also known as restriction fragment length polymorphism (RFLP) and enterobacterial repetitive intergenic consensus (ERIC)‐PCR (Rafiee et al., 2000).
Table 1: Clinical and pathological outcome from experimental inoculation with strains from different serovars of H. parasuis (Straw et al., 2013).
3. Epidemiology
H. parasuis is a member of the normal respiratory microbiota and is ubiquitous in swine herds worldwide. Colonization of the upper respiratory tract of piglets by H. parasuis occurs soon after birth through contact with the sow. However, H. parasuis is not always detected in the nasal cavity of dams, probably due to low quantity of bacteria in these animals. Attempts to isolate H. parasuis from the vagina of the sows have failed. This is in agreement with the production of H. parasuis‐free piglets by snatch farrowing and subsequent artificial feeding. Snatch‐farrowed colostrum‐deprived piglets have been useful to experimentally reproduce disease with H. parasuis (Oliveira et al., 2003).
H. parasuis has been detected in nasal swabs of piglets with a maximum prevalence of colonization occurring at 60 days of age. Different strains of
H. parasuis can be isolated from the nasal cavities of piglets, and a single animal can carry more than one strain. Four to five strains can be isolated from a herd at a given time, and up to 16 different strains were isolated in a single farm during one production cycle. In spite of the variety of strains within a herd, usually one prevalent strain is associated with an outbreak (Rafiee et al., 2000)
3.1 Transmissions
Transmission of H. parasuis occurs through contact of carrier or diseased pigs with susceptible animals. Thus, mixing pigs from different origins and ages is a risk factor for transmission. H. parasuis is very labile in the environment. Although there are not many studies on its resistance to disinfectants, efficacy of several formulations, including chloramine‐T and quaternary ammonium compounds, has been reported (Ferri et al., 2010).
4. Pathogenesis
H. parasuis pathogenesis requires entry into the host, evasion of host defenses, bacterial multiplication, and damage to tissues (Figure 1). Disease caused by H. parasuis has been reproduced with virulent strains by different inoculation. After intranasal inoculation H. parasuis was detected in the nasal mucosa where suppurative rhinitis and epithelial cell degeneration were observed. Induction of adhesins and biofilm formation may play an important role at this stage, especially in the normal colonization by non‐virulent strains. Bacterial adhesion to and invasion of epithelial cells, induction of apoptosis, and cytokine release can also be important events for H. parasuis colonization. CDT and sugar residues of the lipooligosaccharide (LOS) are involved in the interaction with epithelial cells. After nasal colonization, the bacterium is isolated from lung and later only virulent strains from internal organs after a short passage through blood. In the lung, virulent strains of H. parasuis survive the phagocytic activity of porcine alveolar macrophages (PAMs) and can be detected in the lung tissue by immunohistochemistry (IHC). In contrast non‐virulent strains are efficiently cleared from the lung by PAMs and are retained in the upper respiratory tract. Macrophage activation is delayed by virulent strains, and lung infection is established. Phagocytosis resistance is likely associated with the expression of capsule, as well as with other genes, including two virulence‐associated trimeric autotransporters (vtaA), VtaA8 and VtaA9. In agreement, several genes with homology to putative virulence factors, such as several vtaAs and siaB (involved in sialic acid utilization), or a protease, were expressed in vivo in the infected lung. Survival in the lung by virulent strains is accompanied by changes in bacterial metabolism, including upregulation of iron uptake genes. Besides, H. parasuis possess a neuraminidase that operates as scavenger of sialic acid for nourishment or modification of the bacterial surface to evade the immune system (Perry et al., 2013)
H. parasuis invades endothelial cells and induces apoptosis and production of proinflammatory interleukin‐6 (IL‐6) and interleukin‐8 (IL‐8). These phenomena may play a role in the passage to the blood and across the blood–brain barrier. LOS has a partial role in endothelial adhesion and induction of. Virulent strains are able to survive the bactericidal effect of the blood complement and can reach systemic sites. Several bacterial factors are involved in serum resistance, including the polysaccharide biosynthesis protein and the outer membrane protein P2. Systemic spread of H. parasuis induces a severe increase in proinflammatory IL‐8 and soluble CD163 in blood, with the subsequent inflammation and production of the characteristic lesions of Glässer’s disease in serosae. In the spleen of infected pigs, genes involved in immune response were differentially expressed and included inflammatory molecules, acutephase proteins, adhesion molecules, complement, and genes with functions in antigen processing and presentation (Chen et al., 2009).
Severity of disease depends on the virulence of the H. parasuis strain, the immunity of the piglets, the concomitant presence of other pathogens in the herd, and the genetic resistance of the host. H. parasuis can act as primary or secondary pathogen. Immunosuppressive events, such as viral infections that alter the immune system, allow H. parasuis strains that are usually restricted to the respiratory tract to invade and be isolated from systemic sites. An epidemiological association between H. parasuis infection and PRRSV, porcine circovirus type 2 (PCV2), and influenza virus has been reported. Exacerbation of disease by H. parasuis with PRRSV was experimentally confirmed by concurrent infection with both agents, although earlier studies failed to find such association. A work by Brockmeier (2004) linked higher nasal colonization by H. parasuis to prior colonization by Bordetella bronchiseptica. The influence of genetic background on resistance to H. parasuis systemic infection has been evaluated. Blanco et al. (2008) reported that piglets obtained from six different sire boars significantly varied regarding their resistance, which was associated with higher levels of antibacterial activity in the lung. Some genetic markers (BAT2, Mx1, and EHMT2 variations) have been also associated with the risk of infection by H. parasuis (Wang et al., 2012).
Even though domestic pig and wild boar are the only natural hosts for H. parasuis, the guinea pig model is suitable to reproduce lesions, and the mouse model has been proven useful to study the protective capacity of experimental vaccine candidates (Zhou et al., 2009).
Figure 1: Main events in H. parasuis pathogenesis. Virulent strains delay activation of alveolar macrophages, survive in the lung, and invade systemic organs, while non‐virulent strains are cleared from the lung by alveolar macrophages and remain in the upper respiratory tract. Invasion of virulent strains induces severe inflammation and the characteristic lesions of Glässer’s disease. Blue represents some virulence factors (Straw et al., 2013).
a Sugar residue of the capsular lipooligosaccharide (LOS) and bacterial cytolethal distending toxin (CDT) are important in adhesion to host epithelial cells
b Bacterial capsule and two virulence-associated trimeric autotransporters (VtaA8 and VtaA9) interfere with phagocytosis by host leukocytes
c Pulmonary alveolar macrophages (PAMs)
d During tissue invasion, the number of activated macrophages increases with an associated increase in proinflammatory cytokines sCD163 and interleukin-8
5. Clinical signs and lesions
5.1 Clinical signs
Clinical signs are mainly observed in 4‐ to 8‐week‐old pigs, although the age of affected animals may vary, depending on the level of acquired maternal immunity and colonization. Also, Glässer’s disease cases can be sporadically observed in adult pigs. Based on experimental infections using H. parasuis‐free piglets (Aragon et al., 2010), the incubation period varies depending on the infecting strain and ranges from less than 24 hours to 4–5 days post inoculation.
Peracute disease has a short course (<48 hours) and may result in sudden death without characteristic gross lesions. The typical clinical signs of acute Glässer’s disease include high fever (41.5 °C), coughing, abdominal breathing, swollen joints with lameness, and central nervous signs such as lateral decubitus, paddling, and trembling. These signs may be seen jointly or independently. Animals with mild to moderate clinical signs usually survive the acute phase of disease and may develop a chronic stage characterized by rough hair, reduced growth rate, and lameness. Dyspnea and coughing have also been described together with H. parasuis isolation from lungs with cranioventral consolidation. Other very sporadic clinical outcomes have been associated with H. parasuis infection, such as acute myositis of the masseter muscles in gilts.
Morbidity and mortality rates associated with Glässer’s disease in affected farms have been traditionally very variable, but they usually vary from 5 to 10% in conventional farms. Concomitant stressors, mainly viral infections that alter the immune system, also modulate disease prevalence. As stated above an increase of Glässer’s disease prevalence has been described in farms affected by other pathogens, such as PRRSV, PCV2, and influenza (Straw et al., 2013).
5.2 Lesions
Peracutely diseased pigs usually die without characteristic gross lesions but may show petechial hemorrhages in some tissues. Histologically, those pigs show septicemia‐like microscopic lesions such as disseminated intravascular coagulation and micro‐hemorrhages (Amano et al., 1994). Increased serosanguinous fluid in the thoracic and abdominal cavities, without fibrin, can also be seen in peracute cases of H. parasuis infection. Acute systemic infection is characterized by the development of fibrinous or fibrinopurulent polyserositis, polyarthritis, and meningitis (Figure 2). The fibrinous exudate can be observed on the pleura, pericardium, peritoneum, synovia, and meninges and is usually accompanied by an increased amount of fluid. Fibrinous pleuritis may be found with or without cranioventral consolidation due to catarrhal purulent bronchopneumonia. Lack of characteristic gross lesions is also common in a number of pigs showing neurological clinical signs. Histopathological examination of typical Glässer’s disease reveals fibrinous to fibrinopurulent serositis and usually does not contribute additional useful information to the diagnosis except the detection of potential fibrinopurulent meningitis (Figure 2c). Recently, pyelitis has been described as a consistent histopathological finding in experimentally infected conventional pigs with H. parasuis serovar 5 (Palzer et al., 2015). Chronically affected animals usually show severe fibrosis of the pericardium, pleura, and/or peritoneum, as well as chronic arthritis.
6. Diagnosis
6.1 Differential diagnosis
The clinical signs and lesions described for H. parasuis systemic infection are not pathognomonic of this agent, and other agents must be included in a differential diagnosis. Fibrinous polyserositis may be caused by other gram‐negative bacteria such as nonhemolytic Escherichia coli. Polyserositis caused by nonhemolytic E. coli is sporadic and usually affects suckling piglets (Nielsen et al., 1975). Beta‐hemolytic Shiga toxin 2e (Stx2e) ‐positive E. coli can cause central nervous system signs in recently weaned pigs (edema disease) similar to those observed with H. parasuis systemic infection. However, these pigs do not develop fibrinopurulent meningitis typical of H. parasuis. Mycoplasma hyorhinis is another important cause of fibrinous polyserositis in nursery pigs, and it is frequently found coinfecting pigs with H. parasuis. Streptococcus suis usually affects pigs at the same age as H. parasuis and may cause similar lesions. Other agents involved in development of lameness and arthritis include Erysipelothrix rhusiopathiae and Mycoplasma hyosynoviae. However, both agents are more likely to cause chronic non‐purulent arthritis in finishing pigs. Considering that H. parasuis is a colonizer of the upper respiratory tract of healthy pigs, detection of this microorganism in the nasal cavity and trachea does not imply disease development. Systemic isolates are more likely to carry important virulence factors, and, therefore, those isolates should be pursued for laboratory confirmation of morbidity and mortality caused by this agent. H. parasuis can be isolated from the fibrinous exudate and parenchyma of affected internal organs and from lung lesions in pneumonia cases. It is recommended to take several samples from the same animal (Straw et al., 2013).
6.2 Isolation
H. parasuis is a fastidious microorganism with limited survival at room temperature and special requirements for in vitro growth. The chances of isolating H. parasuis can be considerably improved by utilizing swabs with Amies transport media and submitting samples under refrigeration to the laboratory (Del Río et al., 2003). Although H. parasuis isolation can be a difficult task, it is important to obtain an isolate for antibiotic sensitivity assessment and typing.
6.3 PCR
PCR is a sensitive and specific method to detect H. parasuis. Several methods are described in the literature, varying from conventional gel‐based tests to realtime detection. PCR detects H. parasuis even when the organism is no longer viable, and it represents a great advantage in sensitivity compared with bacterial isolation. A real‐time PCR showed a detection limit of 0.83–9.5 CFU per reaction and was more sensitive and specific than previously reported gelbased methods (Olvera et al., 2012). PCR can be used also for differentiation between virulent and non‐virulent isolates. A multiplex test based on the detection of a fragment of vtaAs can be used to further characterize isolates regarding invasiveness potential. This information is relevant, especially when selecting isolates for antibiotic sensitivity or autogenous vaccine production (Straw et al., 2013).
6.4 ELISA
ELISA can detect antibodies against H. parasuis, although this technique has mostly been used for research purposes. Whole cell indirect ELISAs have been used to characterize the transfer of maternal immunity from sows to piglets and to demonstrate seroconversion post‐vaccination. Blanco et al. (2004) utilized a commercial ELISA to characterize the decay of maternal antibodies and identify a window of susceptibility in conventional pigs for experimental infection. The specificity of experimental and commercially available H. parasuis ELISAs has not been extensively evaluated, and their ability to detect antibodies against different H. parasuis serovars and strains has been poorly characterized (Straw et al., 2013).
7. Treatment
H. parasuis is one of the few gram-negative organisms that can be successfully treated with synthetic penicillin. Other antimicrobials used include ceftiofur, ampicillin, enrofloxacin, erythromycin, tiamulin, tilmicosin, florfenicol, and potentiated sulfonamides. Individual treatments must be given parenterally to see a significant effect, and all pigs in affected groups (not just those showing clinical signs) will eventually require treatment. Preventive treatments should be discouraged to avoid development of resistance to antimicrobials (Straw et al., 2013).
8. Control and prevention
8.1 Antibiotics
Antibiotics are widely used to prevent and control H. parasuis disease, but increasing pressure to reduce reliance on antibiotics, particularly for population‐level prophylaxis, puts more emphasis on vaccination strategies to prevent systemic infection and mortality. Antibiotic treatment remains an essential control measure in the face of severe outbreaks of H. parasuis systemic infection. Injectable treatments tend to be more effective than water or feed medication, since pigs clinically affected by H. parasuis systemic infection are less likely to ingest the required antibiotic dose via food and water intake. Nonsteroidal anti‐inflammatory treatment may assist recovery. Antibiotic susceptibility profiles are variable in different countries and reflect the selection of drugs used in each region. For example, Danish, British, and Australian isolates are reported as broadly susceptible to most antibiotics, whereas high rates of resistance to commonly used antibiotics are reported for Chinese and Spanish isolates. Resistance to tetracycline and beta‐lactam antibiotics has been linked to the presence of resistance genes in plasmids carried by H. parasuis. Recent data indicate that low variability in the composition of the nasal microbiota at weaning, a condition that may be associated with antibiotic use, is a predisposing factor for disease development by H. parasuis (Correa-Fiz et al., 2016).
H. parasuis is a commensal of the upper respiratory tract and colonizes virtually every pig. The early colonization of pigs with virulent strains in the presence of maternal immunity actually prevents disease and mortality post weaning. However, natural colonization during the suckling period usually is not protective against the wide variety of strains circulating in different swine populations owing to poor heterologous protection. Therefore, commingling of pigs from different sources and ages is an important cause of increased mortality due to Glässer’s disease and should be avoided when possible. Although elimination of H. parasuis from a herd is difficult due to early maternal colonization of neonatal piglets, it may be achieved through Cesarean derivation into pig‐free premises. However, except in circumstances where standards of biosecurity are exceptional, elimination is not recommended because such herds are highly susceptible to severe outbreaks if exposed to virulent H. parasuis due to a lack of immunity. Strict biosecurity that forbids introduction of H. parasuis‐positive swine or fomites is essential to prevent high mortality in such cases.
8.2 Vaccination
Vaccination is an effective measure to prevent mortality. Commercially available bacterin vaccines are based on serovar 5, combined serovars 4 and 5, or combined serovars 1 and 6 for use in sows and pigs. All these products show limited cross‐protection to heterologous strains. Studies have failed to show interference from maternal antibody where sow and piglet vaccination are practiced concurrently in acute disease contexts. More recently, a live attenuated serovar 5 strains has become available in North America with cross‐protection claimed for serovars 4 and 13. Autogenous vaccines are highly effective in protecting susceptible pigs (McOrist et al., 2009), but it is essential to use typing methods to ensure that the appropriate systemic isolate is incorporated into the vaccine preparation by sampling from systemic rather than respiratory locations.
9. References
Amano, H., Shibata, M., Kajio, N., Morozumi, T., 1994. Pathologic observations of pigs intranasally inoculated with serovar 1, 4 and 5 of Haemophilus parasuis using immunoperoxidase method. Journal of Veterinary Medical Science 56, 639-644.
Angen, O., Oliveira, S., Ahrens, P., Svensmark, B., Leser, T.D., 2007. Development of an improved species specific PCR test for detection of Haemophilus parasuis. Veterinary Microbiology 119, 266-276.
Aragon, V., Cerdà-Cuéllar, M., Fraile, L., Mombarg, M., Nofrarías, M., Olvera, A., Sibila, M., Solanes, D., Segalés, J., 2010. Correlation between clinico-pathological outcome and typing of Haemophilus parasuis field strains. Veterinary Microbiology 142, 387-393.
Correa-Fiz, F., Fraile, L., Aragon, V., 2016. Piglet nasal microbiota at weaning may influence the development of Glässer’s disease during the rearing period. BMC genomics 17, 1-14.
Chen, H., Li, C., Fang, M., Zhu, M., Li, X., Zhou, R., Li, K., Zhao, S., 2009. Understanding Haemophilus parasuis infection in porcine spleen through a transcriptomics approach. BMC genomics 10, 1-18.
Del Río, M., Gutiérrez, C., Rodríguez Ferri, E., 2003. Value of indirect hemagglutination and coagglutination tests for serotyping Haemophilus parasuis. Journal of Clinical Microbiology 41, 880-882.
Ferri, E.R., Martínez, S., Frandoloso, R., Yubero, S., Martín, C.G., 2010. Comparative efficacy of several disinfectants in suspension and carrier tests against Haemophilus parasuis serovars 1 and 5. Research in veterinary science 88, 385-389.
Howell, K.J., Weinert, L.A., Luan, S.-L., Peters, S.E., Chaudhuri, R.R., Harris, D., Angen, Ø., Aragon, V., Parkhill, J., Langford, P.R., 2013. Gene content and diversity of the loci encoding biosynthesis of capsular polysaccharides of the 15 serovar reference strains of Haemophilus parasuis. Journal of Bacteriology 195, 4264-4273.
Kielstein, P., Rapp-Gabrielson, V.J., 1992. Designation of 15 serovars of Haemophilus parasuis on the basis of immunodiffusion using heat-stable antigen extracts. Journal of Clinical Microbiology 30, 862-865.
McOrist, S., Bowles, R., Blackall, P., 2009. Autogenous sow vaccination for Glasser’s disease in weaner pigs in two large swine farm systems. Journal of Swine Health Production 17, 90-96.
Nielsen, N., Bille, N., Riising, H., Dam, A., 1975. Polyserositis in pigs due to generalized Escherichia coli infection. Canadian Journal of Comparative Medicine 39, 421.
Nielsen, R., 1993. Pathogenicity and immunity studies of Haemophilus parasuis serotypes. Acta Veterinaria Scandinavica 34, 193-198.
Oliveira, S., Galina, L., Blanco, I., Canals, A., Pijoan, C., 2003. Naturally-farrowed, artificially-reared pigs as an alternative model for experimental infection by Haemophilus parasuis. Canadian Journal of Veterinary Research 67, 146.
Olvera, A., Pina, S., Macedo, N., Oliveira, S., Aragon, V., Bensaid, A., 2012. Identification of potentially virulent strains of Haemophilus parasuis using a multiplex PCR for virulence-associated autotransporters (vtaA). The Veterinary Journal 191, 213-218.
Palzer, A., Austin-Busse, R.-L., Ladinig, A., Balka, G., Zoels, S., Ritzmann, M., 2015. Histopathologic lesions in conventional pigs experimentally infected with Haemophilus parasuis serovar 5. Tierärztliche Praxis Ausgabe G: Großtiere/Nutztiere 43, 91-96.
Perry, M.B., MacLean, L.L., Gottschalk, M., Aragon, V., Vinogradov, E., 2013. Structure of the capsular polysaccharides and lipopolysaccharides from Haemophilus parasuis strains ER-6P (serovar 15) and Nagasaki (serovar 5). Carbohydrate research 378, 91-97.
Rafiee, M., Bara, M., Stephens, C., Blackall, P., 2000. Application of ERIC PCR for the comparison of isolates of Haemophilus parasuis. Australian Veterinary Journal 78, 846-849.
Segalés, J., 2020. Glasser disease in pigs. MSD Manual. Veterinary Manual.
Straw, B.E., Zimmerman, J.J., D'Allaire, S., Taylor, D.J., 2013. Diseases of swine. John Wiley & Sons.
Wang, S., Liu, W., Yang, L., Liu, H., Sargent, C., Affara, N., Zhang, S., 2012. Association of multi-pathogenic infections with BAT2, CXCL12, Mx1 and EHMT2 variations in pigs. Molecular Biology Reports 39, 8169-8176.
Zhou, M., Guo, Y., Zhao, J., Hu, Q., Hu, Y., Zhang, A., Chen, H., Jin, M., 2009. Identification and characterization of novel immunogenic outer membrane proteins of Haemophilus parasuis serovar 5. Vaccine 27, 5271-5277.
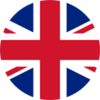