To My Quyen,
Nguyen Khanh Thuan, Nguyen Phuc Khanh, Nguyen Thanh Lam*
1. Introduction
Haemonchosis is one of the most significant parasitic diseases of livestock worldwide, which is a main cause of production losses and ill-health in sheep and goats. This disease is caused by Haemonchus contortus (H. contortus), a nematode parasite of the ruminants’ abomasa. It is one of the greatest pathogenic nematodes, known as ‘barber’s pole worm or red stomach worm or wire worm’ of small ruminants, which inhabits the abomasum. The acute and emaciated form of disease is commonly seen in young animals, while adult animals are more resistant to infestation (Onyenwe et al., 2005). The common clinical signs are ascites, weight loss, anaemia, sub-mandibular edema, and death. Infection with H. contortus caused a significant reduction in the erythrocyte counts, haemoglobin concentrations and packed cell volume (PCV) and significant increased white blood cells (WBC) and eosinophil, also produce significant reduction in total protein, calcium and iron (Alam et al., 2020).
2. Aetiology
2.1 Bacterial characteristics
Macroscopically, H. contortus worms were easily seen even amongst the debris in opened abomasum, it is a bright red colour. The female has white ovaries and uteri were twisted spirally around the red blood-filled intestine (barber’s pole or wire appearance). The male was measured 15–20 mm, while the female 24–30 mm in length. Microscopically, the anterior end showed a small buccal capsule containing small lancet and a pair of wedge-shaped cervical papillae. The male bursa was well developed and consisted of two lateral lobes, it was supported by a characteristic Y-shape dorsal ray, and the spicules were long, equal, brownish in color, and end with barbed tips. The female vulva was covered with flap (Linguiform or knobbed) or not (smooth) (Figure 1) (Onyenwe et al., 2005).
2.2 Classification
The relationship of H. contortus phosphoinositide 3-kinase (PI3K) catalytic subunit Hc-AGE-1 and regulatory subunit Hc-AAP-1 was conducted by subjecting to phylogenetic analyses (Figure 2a). There was concordance in topology among the MP, ML and NJ trees, which all displayed three strongly supported clusters representing classes I, II and III, respectively. Hc-AGE-1 had the closest relationship to class I homologs from C. elegans and C. briggsae. The predicted amino acid sequence of Hc-AAP-1 was aligned with ten AAP-1 homologues (from eight nematodes and two other metazoans) and subjected to phylogenetic analyses (Figure 2b).
Again, there was concordance in topology among the NJ, MP and ML trees which showed that Hc-AAP-1 has its closest relationship with Caenorhabditis homologs, to the exclusion of AAP-1 s from S. stercoralis as well as A. suum,
B. malayi and L. loa, with the AAP-1 s from the latter three species grouping together with absolute modal support (100%). Interestingly, AAP-1 of Trichinella spiralis grouped with D. melanogaster and H. sapiens homologues, with 97% support (Figure 2b) (Li et al., 2014).
3. Epidemiology
A warm, moist environment is needed for the free-living stages of H. contortus to survive and develop outside the host. If suitable conditions are present in any season, haemonchosis can occur (Waller et al., 2004). In the tropical climate zones between 23.5° North and South, such as South-East Asia, Southern India, Central Africa, and America and Northern South America, H. contortus survives well and disease prevalence is highest. Sub-tropical regions immediately north and south of 23.5° N and 23.5° S, including parts of Australia, Southern Africa, Southern North America, South America, and South-eastern China have warm, humid, summer-dominant rainfall, providing suitable environments for H. contortus survival. Warm temperate zones beyond 40° latitude in New Zealand, Northern Europe, Scandinavia, North Asia, and North America have suitable conditions in wet summers and autumns for larval survival, while temperate zones between 45° and 65° latitude in Sweden, France, Denmark, and the Netherlands are too cool most of the year to permit larval survival and development. Haemonchosis is rare in arid regions of the world as there is insufficient moisture for the free-living larval stages of H. contortus. However, increased rainfall or irrigation can allow larvae to survive in warmer arid areas. Additionally, important is the ability of the fourth larval stage of H. contortus to undergo hypobiosis (arrested development), contributing to their ability to survive in cold or arid environmental conditions (Flay et al., 2022).
Optimal conditions for H. contortus egg hatching and larval development occur at ambient vegetation microclimate temperatures of 22 to 26 °C and humidity close to 100%. During dry weather, larvae may remain in desiccated faeces and emerge after rain, contributing to a surge in infection. If ideal environmental conditions are present, development from the egg to infective third stage larvae (L3) can occur within four days, otherwise, larval development time is more variable. Low humidity and desiccation rapidly kill both eggs and larvae (Getachew et al., 2007).
4. Genomic and proteomic profile exploited from H. contortus
During the last two decades, deep insights into the molecular biology of the most significant parasitic nematode H. contortus have explored a large number of novel vaccine components by utilizing genomic and proteomic tools. Genome-wide transcriptomic data from all the key developmental stages of H. contortus revealed 23,610 protein-coding genes involved in host–parasite interactions, development, reproduction, immunity, and disease (Yatsuda et al., 2003). A comprehensive proteomic analysis using matrix-assisted laser desorption ionization time-of-flight mass spectrometry (MALDI-TOFMS or MALDI-TOF-MS/MS) and liquid chromatography tandem mass spectrometry (LC-MS/MS) pointed out 107 identities from 102 spots, including novel groups of proteins, such as aminopeptidases (H11), zinc metalloproteases, serine and aspartic proteases, and the most important immunogenic vaccines components of H. contortus ESPs (HcESPs) such as Hc-15, Hc-24, Hc-40, and apical gut proteins (GA1). Whole protein extracts from male and female H. contortus identified 23 immunogenic, 132 female-specific, and 129 male-specific proteins of adult H. contortus parasite. The most recent identification of the secretome from L3s, L4s, and adult male and female developmental phases (stages/sexes) was carried out by LC-MS/MS analysis, in which 878 novel ES proteins were identified mainly involved in acquisition, nutrient digestion, and host–parasite interactions (Wang et al., 2019).
A study that was conducted by Roz Laing et al. (2020) used extensive transcriptomic evidence from across the H. contortus lifecycle. It is predicted that a similar number of protein-coding genes to the C. elegans genome (21,799 versus 20,532), but significantly lower gene density of 59 genes per Mb, with only 7% of the genome being protein coding, compared to 200 genes per Mb and a protein coding content of approximately 30% in the C. elegans genome (Figure 3). The average coding sequence length is similar in H. contortus and C. elegans (1,127 bp in H. contortus compared to 1,371 bp in C. elegans) but the average gene length is more than double in the parasite (6,564 bp compared to 3,010 bp in C. elegans). A closer look at a subset of 2,822 H. contortus and C. elegans one-to-one orthologs shows the discrepancy is explained by an expansion in both the number and length of introns in H. contortus (average of 10 introns per gene, average size 633 bp; relative to 6 introns per gene, average size 340 bp in C. elegans(Dieterich et al., 2008).
5. Life cycle
The life cycle of H. contortus starts when adult male and female worms reproduce sexually in the host abomasum with an impressive output per female worm estimated from 5,000 to 15,000 eggs/day.
The eggs embryonate into first-stage larvae (L1) and second-juvenile stage larvae (L2) within four to six days and start feeding on bacteria in the dung. Under optimal conditions of 24–29°C, the L2 larvae moult into the third stage (L3), but they retain the cuticle from the previous moult. During grazing, the infective stage L3 larvae reach the abomasum, shed their cuticle, and burrow into the internal layer of the abomasum and ex-sheath into L4 (pre-adult) stage, usually within 48 h, and finally develop to early (L5) and then late adult worms, which start feeding on blood (Figure 4). During the infective L3 or early L4 larval stages, parasites do not proceed directly to the next stage; instead, they remain in the gastric glands of the abomasum. This phenomenon is called developmental arrest (hypobiosis/diapause), and it is described as a “developmental stage in which the parasite becomes dormant, does not cause disease, and metabolically remains inactive”. If the conditions outside the host are unfavorable for parasite development, the portion of hypobiotic worms is usually higher so that any egg shed into the environment would be unlikely to develop and survive. This common mechanism of many parasitic worms, including H. contortus, depends on external factors and the immune responses of hosts as well, and also the genes involved in this phenomenon might serve as key antigens for new drug/vaccine targets in the control and prevention of H. contortus infection (Ehsan et al., 2020).
5. Pathogenesis
5.1. Parasitic stages of H. contortus
After ingestion by susceptible sheep, the L3 differentiate, and by the third day after infection, complete the first parasitic ecdysis (cuticle shedding), becoming the fourth larval stage (L4). By days seven to nine, the L4 can be up to 5 mm in length and a second ecdysis has taken place, forming the fifth larval stage (L5). Around days ten to eleven, just before the final ecdysis, the immature L5 develop a lancet in their buccal cavity and burrow through the mucous layer on the surface of the abomasum into the gastric pits of the abomasal mucosa. Once in the gastric pits, the L5 use the lancet to cut the abomasal tissue and induce haemorrhages before ingesting the leaking blood (Tehrani et al., 2012). The L5 larvae then mature into adult forms, continue feeding, and begin sexual differentiation and reproduction. Adult H. contortus females begin to produce eggs from day 12 to 15 and have a high biotic potential (Flay et al., 2022).
5.2. Syndromes of H. contortus
5.2.1. Hyperacute Haemonchosis
Hyperacute haemonchosis is rare and occurs in animals exposed to heavy burdens of H. contortus (up to 30,000 larvae per animal) over a short period of time. It is more common in young animals. Death is sudden with either no previous clinical signs, or anaemia and melaena may be detected in surviving animals (Besier et al., 2016).
In lambs, haemoglobin concentrations below 30 g/L have been associated with mortality. Infected sheep can lose 0.2–0.6 L of blood per day, an approximate equivalent of 18–54 g of haemoglobin per day and can exsanguinate within a week before compensatory erythropoiesis occurs. The volume of blood lost in this time may represent half of the blood volume of a lamb. Although the pathogenesis of death for hyperacute haemonchosis has not been clearly described, it is reasonable to assume that death can be attributed to hypovolemic shock. Severe haemorrhage causes a rapid decrease in the circulating blood volume and blood pressure, culminating in hypovolemic shock where tissue perfusion is insufficient to sustain aerobic metabolism (Gutierrez et al., 2004).
5.2.2. Acute Haemonchosis
Acute haemonchosis is characterized by lower burdens of H. contortus (approximately 2000–20,000 larvae per animal), resulting in a less severe loss of blood and anaemia that develops over a longer period of time, compared to hyperacute haemonchosis. In acute haemonchosis, sheep show clinical signs associated with anaemia and hypoproteinemia such as lethargy, weakness, increased respiratory and heart rates, and pale mucous membranes. There is an initial decrease in PCV, but compensatory erythropoiesis occurs within the first 14 days of infection, and full compensation and apparent recovery is seen over a period of six weeks after infection (Flay et al., 2022).
In most mammals, including ruminants, compensatory erythropoiesis can be detected three to four days after the onset of haemorrhage or haemolysis. Compensatory erythropoiesis continues until the animal’s baseline PCV is reached. A threefold increase in the rate of erythropoiesis and iron utilization has been described in sheep infected with H. contortus. It can be assumed, therefore, that this compensatory process may result in an initial steady normalization of the infected sheep’s PCV as was described. However, the continuous loss of blood over a period of weeks outpaces iron reabsorption in the intestine, with the progressive depletion of iron stores leading to a state of iron deficiency (Dargie and Allonby, 1975).
Iron deficiency can result from insufficient intake, inadequate absorption of iron from the intestinal tract, or chronic external blood loss. Iron deficiency is defined as the reduction in total body iron and may progress over three sequential stages. When bone marrow iron stores are depleted and transport iron is low, iron-deficient RBC are produced, and the PCV drops but remains within normal limits. In the third stage, there is iron deficiency anaemia. The PCV drops below normal limits, and initially, the anaemia is regenerative. However, as the iron deficiency worsens, a microcytic hypochromic anaemia manifests, and the regeneration becomes inadequate for the degree of anaemia (Bergmann et al., 2021).
Haemonchosis causes concurrent hypoproteinemia. Hypoalbuminemia results in decreased intravascular oncotic osmotic pressure and oedema that may be generalized or localized in the intermandibular space or cervical region. Oedema can form in any organ or tissue, but it is most commonly seen in subcutaneous tissue and more obvious in regions with high hydrostatic pressures, or in lower parts of the body related to the influence of gravity. These factors may explain the formation of oedema in the intermandibular space seen in acute haemonchosis, although some sheep may die before the development of oedema (Flay et al., 2022).
5.2.3. Chronic Haemonchosis
In chronic or subclinical haemonchosis, sheep ingest lower numbers of H. contortus and infections may go unnoticed. Chronic haemonchosis typically occurs in environments not suitable for the development of the infective larvae during less favourable periods in seasonally endemic zones, or where there are effective control measures to prevent acute haemonchosis. Infection may manifest as a reduction in productivity, feed conversion, growth rate, or milk yield (Cobon and O'Sullivan, 1992).
6. Clinical signs and pathology
6.1 Clinical signs
The infected animals also showed various clinical signs, including diarrhea, weakness and debilitation, anemia, and weight loss (Figure 6). Some goats showed submandibular edema (bottle jaw). Some animals experienced hemorrhage on the mucosa of the abomasa, and worms were detected during postmortem examination (Figure 5). Furthermore, the occurrence of hemonchosis in the examined animals was significantly affected by the age and sex of the animals and season. Goats aged 1 year or older were at a higher risk than those younger than 1 year. Regarding sex, males were more affected by hemonchosis than females (Gareh et al., 2021).
6.2 Pathology
Macroscopic findings
Harbouring variable number of worms, the abomasum was endowed with gross lesions such as thickening of mucosal folds, petechial haemorrhages and nodule development which were more visible on the fundic region in both infected sheep and goats (Fig. 7). Such lesions were more prominent in goats than in sheep (Abosse et al., 2022).
(A) large number of adult worms; (B) thickened and rough mucosal folds (arrow), (C) nodular lesion (blade) and petechial haemorrhages (arrow) in the fundic region.
Microscopic findings
Histological examination of the fundic region of the non-infected abomasums revealed intact surface epithelium with goblet cells and glandular structure with few leukocyte populations in the lamina propria and submucosa (Fig. 8). On the other hand, denuded surface epithelium, damaged gastric pits, hemorrhagic submucosal layer as well as a cross-section of tissue doweling immature worm were observed at low magnification in infected sheep and goats (Fig. 9). At higher magnification, degenerating chief and parietal cells, as well as very high cellular infiltration dominated by eosinophils were a common characteristic of infected abomasal. Tissue eosinophilia was more extensive in sheep both in terms of distribution along the different layers of the mucosa and the number of cells under a single field of vision (Fig. 10) (Abosse et al., 2022).
7. Diagnosis
Antemortem methods for confirmation of H. contortus infections include testing for anaemia and confirming the presence of parasites. While detection of H. contortus by faecal egg counts and larval culture are a conventional method for diagnosis, by the time egg-laying adults develop, affected sheep can already be anaemic (Flay et al., 2022).
The total blood volume of a lamb at birth has been determined to be 0.17 L per kilogram of bodyweight, dropping to about 0.1 L/kg by day 30 of age. A decrease in the concentration of RBC, haemoglobin, and PCV can all be defined as anaemia. The normal haematological reference range values for sheep are listed in Table 1 (Flay et al., 2022).
Table 1:
Normal haematological reference range values for sheep (Jackson and Cockcroft, 2008)
Value | Abbreviation | Reference Range | Unit |
Haemoglobin | Hb | 90–150 | g/L |
Haematocrit (packed cell volume) | PCV | 0.27–0.45 | L/L |
Red blood cells | RBC | 8.0–18.0 | ×1012/L |
Mean cellular volume | MCV | 28.0–40.0 | fL |
Mean cellular haemoglobin | MCH | 8.0–12.0 | pg |
Mean cellular haemoglobin concentration | MCHC | 310–340 | g/L |
7.1 Haematology and selected biochemical findings
A range of changes may be seen on a complete blood count (CBC). Anaemia may be mild to severe and regenerative to non-regenerative. With compensatory erythropoiesis (as seen in acute haemonchosis), the anaemia may be macrocytic and hypochromic. With chronic blood loss, the anaemia may become microcytic, hypochromic, and non-regenerative due to iron deficiency. The leukocyte changes are variable; an inverse relationship between worm burden and total leukocyte count has been demonstrated. Lymphopenia is common, but the total leukocyte count may be within normal limits or increase due to an increase of neutrophils and eosinophils. Leukopenia characterized by lymphopenia and neutropenia may occur and may be secondary to migration of the leukocytes into the abomasum where the H. contortus and lesions are located, or due to immune suppression (Alam et al., 2020).
Serum iron and protein concentrations both decreases. Early investigations of the loss of RBC using radioisotopes of iron (Fe59) showed parasitised sheep lost more iron than non-parasitised sheep. The hypoproteinemia may be characterized by low albumin and globulin. This can be attributed to blood loss, protein loss, decreased protein intake, and protein catabolism. Serum protein losses of 10–12 g/L have been recorded in experimental H. contortus infections, from pre-infection concentrations of 62 g/L down to 50 g/L, 20 weeks after infection. Albumin is the principal protein lost, while globulin concentrations may remain in the normal range. This may be due to a combination of the selective loss of the smaller molecule albumin and an increase in globulin, as immunoglobulins are produced in response to inflammation caused by the parasite infection and tissue damage (Bordoloi et al., 2012).
Serum immunoglobulins IgG and IgM may be decreased in sheep with heavy burdens of H. contortus worms. Experimentally infected sheep with heavy worm burdens show significantly lower IgG (9.36 ± 0.16 g/L) and IgM (0.59 ± 0.01 g/L) concentration compared to controls (IgG 10.35 ± 0.09 g/L, IgM 0.83 ± 0.01 g/L) and sheep with light (IgG 10.22 ± 0.05 g/L; IgM 0.74 ± 0.01 g/L) and moderate (IgG 10.12 ± 0.03 g/L; IgM 0.67 ± 0.01 g/L) burdens of H. contortus. This was attributed to translocation of immunoglobulins into the lumen of abomasum to protect against H. contortus (Flay et al., 2022).
7.2 FAMACHA Score
A practical method allowing for anaemia classification in the field was developed in South Africa, based on conjunctival mucous membrane colour in small ruminants [60]. The FAMACHA system (named after the developer Dr Faffa Malan (FAffa MAlan CHArt)) was developed by comparing the colour of the conjunctiva (on a scale of 1–5 with various stages of anaemia related to haemonchosis), with their measured PCV [60]. A value of 1 (red) is considered non-anaemic and a PCV of 35%, 2 a PCV of 25%, 3 a PCV of 20%, 4 a PCV of 15%, through to a value of 5 (white) and a PCV of 5%, being severely anaemic (Flay et al., 2022).
7.3 Post-mortem diagnosis
Post-mortem examination of dead sheep is another rapid, accurate method of confirming a diagnosis, as it allows for visualization of the H. contortus parasites in the abomasum of affected animals. The parasites have a characteristic red and white, twisted “barber’s pole” appearance related to the blood-filled intestine spiralling around the white reproductive tract of the female nematodes, as seen in Figure 11 (Flay et al., 2022).
7.4. Molecular tools
Molecular methods for diagnosis are providing new options for testing for the presence of H. contortus but have limitations due to the inhibition effects of faeces on the polymerase chain reaction (PCR) and their accessibility to veterinarians in the field. Development of suitable PCR tests is on-going, with droplet digital PCR showing promise. In a comparison of diagnostic methods, molecular tests had the greatest sensitivity and were able to identify H. contortus to the species level, while faecal egg counts using the McMaster method had a high specificity but a lower sensitivity than molecular methods (Flay et al., 2022).
8. Future control
8.1 Pharmaceutical control
There are several classes of anthelmintic drugs effective against Haemonchus; these include benzimidazoles (e.g., albendazole), imidazothiazoles (e.g., levamisole), macrocyclic lactones (e.g., ivermectin), salicylanides (e.g., closantel), amino-acetonitrile derivatives (e.g., monepantel), sporoindoles (e.g., derquantel). Commercially available preparations may include the above as single active ingredients or in combinations (Arsenopoulos and Fthenakis, 2021).
In recent years, due to the widespread development of anthelmintic resistance of Haemonchus spp., special emphasis has been given to control and prevention of haemonchosis with the application of both targeted treatments and targeted selective treatments. These approaches aim to keep the largest possible numbers of Haemonchus spp. in refugia, thus maintaining the susceptibility of these parasites to anthelmintic drugs. In refugia is defined as the part of the parasitic population that is not exposed to anthelmintic drugs, i.e., individuals at a free-living (non-parasitic) stage of their biological cycle or individuals that live within a host but still not exposed to anthelmintic treatment (Van Wyk et al., 2006).
The distribution of parasites among animals in a sheep/goat farm is not homogeneous. The majority of the helminths are infecting only a few animals; for example, Sréter et al. concluded that 80% of the parasites were detected in 20% to 30% of the animals, while the majority of the animals were infected with only low parasitic burdens (Sréter et al., 1994). Animals with increased parasitic burdens would be responsible for the spread of nematode larvae in natural pasturelands, as well as usually also showing clinical disease. In addition, the presence of parasitic burden among animals in a farm has a repeatability pattern, i.e., the same animals in the farm seem to develop severe parasitic infections, requiring repeated anthelmintic treatments. The principle of targeted selective treatments is based on the administration of anthelmintics exclusively to animals that need them and would benefit from them, in terms of health and productivity, leaving the rest of the animals in the same flock untreated. The expected advantages of this approach would be the delay of development of anthelmintic resistance, due to the lower exposure of parasites to anthelmintic drugs, the reduced residues of anthelmintic drugs in meat and milk of animals and the smaller costs of treatments (Cabaret, 2008). On the other hand, one significant disadvantage of targeted selective treatments is the possibility that some highly infected animals may escape the anthelmintic treatment, resulting to developing clinical diseases and production losses, as well as disseminating the infection (Arsenopoulos and Fthenakis, 2021).
Various criteria have been proposed to select animals for anthelmintic administration, as part of targeted selective treatment. These include parasitological (e.g., increased egg in faecal samples), clinical (e.g., presence of anaemia detected by the FAMACHA system, presence of diarrhoea or low body condition score) or production (e.g., reduced milk production) criteria. After animals for administration of anthelmintics would be selected based on the above criteria, then one could also select animals to remain untreated, taking into account that at least 10% of the infected animals in a farm should remain untreated, in order to maintain Haemonchus spp. in refugia. Leaving a proportion of sheep in a flock untreated was found to be effective in delaying the development of anthelmintic resistance; a proportion as low as 10% of a flock untreated was sufficient to significantly delay developing a anthelmintic resistance. These animals should be allocated within each grazing group, not just across the flock (Arsenopoulos and Fthenakis, 2021).
8.2 Non-chemical means of control
Protocols for control of haemonchosis by non-pharmaceutical means include (a) grazing management, emphasising on rotational grazing, (b) modification of animals’ diet , which directly affects the response of the animals’ immune system to haemonchosis (Strain and Stear, 2001), (c) selection of animals genetically-resistant against Haemonchus spp. and d) administration of various products to animals, e.g., nematophagous fungi, tannins or polyphenolic compounds (Arsenopoulos and Fthenakis, 2021).
8.2.1 Grazing management
Grazing management, cell or rotational grazing, contributes to minimising the intake of the infective larvae of Haemonchus spp. by susceptible sheep and also limiting excessive contamination of pasturelands with parasitic eggs, that way decreasing the risk of infection. Studies on this approach confirmed the beneficial effect of rotational grazing against haemonchosis, despite the necessity for differing schedules in accord to the various environments. A possible failure to controlling the infection through grazing management can be due to a possible lack of awareness for its potential, as well as for practical reasons, as animals’ movements are determined by nutritional availability (Arsenopoulos and Fthenakis, 2021).
8.2.2 Nutritional management
The ability of ruminants to resist parasitic infections when they are kept in good nutritional status (nutritional management) has been confirmed. It has been established that sheep given a low protein diet seem to be more susceptible to Haemonchus spp. infections compared to animals given supplementary protein. Therefore, there is a relation between nutritional management and resistance to haemonchosis, which can be beneficial when it is planned while considering the epidemiology of the helminths and is applied on its own or in combination with other control strategies (Torres-Acosta et al., 2012).
Many plants contain bioactive compounds, which have been associated with reduced parasitic burdens and increased animal production. Among them, polyphenolic compounds, tannins, in particular, are characterised with antiparasitic properties. These can act directly against the helminths at various stages of their life cycles, or they improve the animals' immune response, reducing the shedding rate. However, there are limitations in their use, as they can lead to detrimental nutritional effects, if fed in excessive rates or when protein intake is low. The use of nematophagous fungi was a promising alternative for use against resistant Haemonchus strains. These have the ability to trap and destroy infective larvae of the helminth, after the formation of hyphae. Specifically, the fungal spores fed to the animals, develop during their passage through the gastrointestinal tract of sheep and predate the infective larvae. Among the most widely evaluated fungal species was Duddingtonia.
flagrans, trials with which showed results supporting their use in sheep or goats for control of H. contortus (Arsenopoulos and Fthenakis, 2021).
Sheep of some breeds (e.g., Morada Nova, Red Maasai, Barbados Black Belly) resistant (or even resilient) to haemonchosis can be used as an interesting option to minimise adverse effects of Haemonchus infection. Further, as such animals harbour fewer worms than ones of susceptible breeds, they also excrete a smaller number of eggs with faeces and do not contribute to intense infection of pastures. Finally, these animals can contribute to controlling anthelmintic resistance, as they would require less frequent anthelmintic treatments. The introgression of these resistant breeds, through crossbreeding, could serve as a desirable alternative, but should be done with prudence, to maintain the production traits of the existing flock (Arsenopoulos and Fthenakis, 2021).
In the same direction, the possible selection of individuals, within a certain breed, resistant (or resilient) against Haemonchus spp. can also be an option. These genetic selection strategies can be facilitated by using traits with relatively high heritability (e.g., faecal egg counts [FEC], haematocrit, body condition). For example, immunity against H. contortus expressed as FEC and packed cell volume values is a heritable trait in sheep, and there are differences among different breeds in Haemonchus infection. However, some studies in Merino sheep breeds have concluded that selection for low H. contortus FEC may also lead to lower animal production. The possibility for more accurate and easily used genetic markers has been widely investigated, including quantitative trait loci, algorithms based on haematological parameters, immunological indicators and molecular markers. Currently, there is one genomic test commercially available (Wormstar, Zoetis), by means of which the resistance variation among individual sheep can be assessed. Potentially, in the future, a potential technology could is the development and commercial availability of sufficient numbers of transgenic animals able to resist the infection (Emery et al., 2016).
9.3 Vaccination
Only recently, vaccines have become available against Haemonchus infection. An effective vaccine against H. contortus has been produced for use in sheep, using the latent H11 antigen, which is a protein of the gastrointestinal tract of H. contortus, and H-gal-GP, which were extracted from adult H. contortus. Clinical trials have confirmed the efficacy of the vaccine, which was developed at the Moredun Research Institute in Edinburgh; for example, significant protection against H. contortus in lambs was indicated after repeated administration with three-week intervals, although findings did not confirm full efficacy in ewes after severe experimental infection. The vaccine is now produced in Australia, where it has been licenced for commercial use in late 2014 (Emery et al., 2016).
10. References
Abosse, J.S., Terefe, G., Teshale, B.M., 2022. Comparative study on pathological changes in sheep and goats experimentally infected with Haemonchus Contortus. Surgical and Experimental Pathology 5, 14.
Alam, R.T., Hassanen, E.A., El-Mandrawy, S.A., 2020. Heamonchus Contortus infection in Sheep and Goats: alterations in haematological, biochemical, immunological, trace element and oxidative stress markers. Journal of Applied Animal Research 48, 357-364.
Arsenopoulos, K.V., Fthenakis, G.C., 2021. Haemonchosis: A Challenging Parasitic Infection of Sheep and Goats. 11.
Bergmann, H., Schulz, K., Conraths, F.J., Sauter-Louis, C., 2021. A Review of Environmental Risk Factors for African Swine Fever in European Wild Boar. Animals 11, 2692.
Besier, R., Kahn, L., Sargison, N., Van Wyk, J., 2016. Diagnosis, treatment and management of Haemonchus contortus in small ruminants. Advances in parasitology 93, 181-238.
Bordoloi, G., Jas, R., Ghosh, J., 2012. Changes in the haemato-biochemical pattern due to experimentally induced haemonchosis in Sahabadi sheep. Journal of Parasitic Diseases 36, 101-105.
Cabaret, J., 2008. Pro and cons of targeted selective treatment against digestive-tract strongyles of ruminants. Parasite 15, 506-509.
Cobon, D., O'Sullivan, B., 1992. Effect of Haemonchus contortus on productivity of ewes, lambs and weaners in a semi-arid environment. The Journal of Agricultural Science 118, 245-248.
Dargie, J., Allonby, E., 1975. Pathophysiology of single and challenge infections of Haemonchus contortus in Merino sheep: Studies on red cell kinetics and the “self-cure” phenomenon. International journal for parasitology 5, 147-157.
Dieterich, C., Clifton, S.W., Schuster, L.N., Chinwalla, A., Delehaunty, K., Dinkelacker, I., Fulton, L., Fulton, R., Godfrey, J., Minx, P., 2008. The Pristionchus pacificus genome provides a unique perspective on nematode lifestyle and parasitism. Nature genetics 40, 1193-1198.
Ehsan, M., Hu, R.-S., Liang, Q.-L., Hou, J.-L., Song, X., Yan, R., Zhu, X.-Q., Li, X., 2020. Advances in the Development of Anti-Haemonchus contortus Vaccines: Challenges, Opportunities, and Perspectives. Vaccines 8, 555.
Emery, D.L., Hunt, P.W., Le Jambre, L.F., 2016. Haemonchus contortus: the then and now, and where to from here? International Journal for Parasitology 46, 755-769.
Flay, K.J., Hill, F.I., Muguiro, D.H., 2022. A Review: Haemonchus contortus Infection in Pasture-Based Sheep Production Systems, with a Focus on the Pathogenesis of Anaemia and Changes in Haematological Parameters. Animals 12, 1238.
Gareh, A., Elhawary, N.M., Tahoun, A., Ramez, A.M., El-Shewehy, D.M., Elbaz, E., Khalifa, M.I., Alsharif, K.F., Khalifa, R., Dyab, A.K., 2021. Epidemiological, morphological, and morphometric study on Haemonchus spp. Recovered from goats in Egypt. Frontiers in Veterinary Science, 1201.
Getachew, T., Dorchies, P., Jacquiet, P., 2007. Trends and challenges in the effective and sustainable control of Haemonchus contortus infection in sheep. Review. Parasite 14, 3-14.
Gutierrez, G., Reines, H., Wulf-Gutierrez, M.E., 2004. Clinical review: hemorrhagic shock. Critical care 8, 1-9.
Jackson, P., Cockcroft, P., 2008. Clinical examination of farm animals. John Wiley & Sons.
Laing, R., Kikuchi, T., Martinelli, A., Tsai, I.J., Beech, R.N., Redman, E., Holroyd, N., Bartley, D.J., Beasley, H., Britton, C., Curran, D., Devaney, E., Gilabert, A., Hunt, M., Jackson, F., Johnston, S.L., Kryukov, I., Li, K., Morrison, A.A., Reid, A.J., Sargison, N., Saunders, G.I., Wasmuth, J.D., Wolstenholme, A., Berriman, M., Gilleard, J.S., Cotton, J.A., 2013. The genome and transcriptome of Haemonchus contortus, a key model parasite for drug and vaccine discovery. Genome Biology 14, R88.
Li, F.-C., Gasser, R.B., Lok, J.B., Korhonen, P.K., Wang, Y.-F., Yin, F.-Y., He, L., Zhou, R., Zhao, J.-L., Hu, M., 2014. Exploring the role of two interacting phosphoinositide 3-kinases of Haemonchus contortus. Parasites & vectors 7, 1-12.
Onyenwe, I.W., Onwe, C., Onyeabor, A., Onunkwo, J.I., 2005. Abattoir-Based study of the susceptibility of two naturally infected breeds of goat to Haemonchus contortus in Nsukka Area of Enugu State, Nigeria. Animal Research International 2, 342-345.
Sréter, T., Molnár, V., Kassai, T., 1994. The distribution of nematode egg counts and larval counts in grazing sheep and their implications for parasite control. International Journal for Parasitology 24, 103-108.
Strain, S., Stear, M., 2001. The influence of protein supplementation on the immune response to Haemonchus contortus. Parasite immunology 23, 527-531.
Tehrani, A., Javanbakht, J., Jani, M., Sasani, F., Solati, A., Rajabian, M., Khadivar, F., Akbari, H., Mohammadian, M., 2012. Histopathological study of Haemonchus contortus in Herrik sheep abomasum. J. Bacteriol. Parasitol 3, 144.
Torres-Acosta, J., Sandoval-Castro, C., Hoste, H., Aguilar-Caballero, A., Cámara-Sarmiento, R., Alonso-Díaz, M., 2012. Nutritional manipulation of sheep and goats for the control of gastrointestinal nematodes under hot humid and subhumid tropical conditions. Small Ruminant Research 103, 28-40.
Van Wyk, J., Hoste, H., Kaplan, R., Besier, R., 2006. Targeted selective treatment for worm management—how do we sell rational programs to farmers? Veterinary parasitology 139, 336-346.
Waller, P., Rudby-Martin, L., Ljungström, B., Rydzik, A., 2004. The epidemiology of abomasal nematodes of sheep in Sweden, with particular reference to over-winter survival strategies. Veterinary parasitology 122, 207-220.
Wang, T., Ma, G., Ang, C.-S., Korhonen, P.K., Koehler, A.V., Young, N.D., Nie, S., Williamson, N.A., Gasser, R.B., 2019. High throughput LC-MS/MS-based proteomic analysis of excretory-secretory products from short-term in vitro culture of Haemonchus contortus. Journal of proteomics 204, 103375.
Yatsuda, A.P., Krijgsveld, J., Cornelissen, A.W., Heck, A.J., de Vries, E., 2003. Comprehensive analysis of the secreted proteins of the parasite Haemonchus contortus reveals extensive sequence variation and differential immune recognition. Journal of Biological Chemistry 278, 16941-16951.
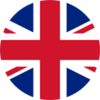