Nguyen Pham Thao Nhi,Nguyen Khanh Thuan, Nguyen Phuc Khanh, Nguyen Thanh Lam*
1. Introduction
Diseases caused by Escherichia coli (E. coli) have been a recognized problem for as long as pigs have been raised. Early work in the 1960s and 1970s elucidated the pathogenic mechanisms of E. coli‐induced neonatal diarrhea and led to the development of sow vaccines that effectively control this form of the disease by passive immunity. However, maternal vaccination does not protect pigs against the diarrhea and edema disease (ED) that occur in the post weaning period.
More recent advances in our understanding of how E. coli causes disease have led to a better classification of pathogenic strains based on the presence of virulence factors, permitting much more accurate diagnostic strategies, as opposed to traditional serotyping identifying the E. coli O and H types. Antimicrobial resistance is at a crisis point for pig producers because of limited treatment options and an increased public health concern due to the potential transfer of drug resistance genes directly by contact and indirectly into the food chain, water, manure, etc. This has provided the impetus to find alternative control strategies such as novel vaccines for weaned pigs (Zimmerman, Karriker et al. 2012).
All over the world, E. coli is an important cause of a wide range of diseases in pigs, including neonatal diarrhea, post weaning diarrhea (PWD), ED, septicemia, polyserositis, coliform mastitis (CM), and urinary tract infection (UTI). In particular, diarrhea and ED due to E. coli may result in significant economic losses due to morbidity, mortality, decreased weight gain, and cost of treatment, vaccinations, and feed supplements. E. coli PWD is also called post weaning enteric colibacillosis. Edema disease is also known as “bowel edema” or “gut edema” because edema of the submucosa of the stomach and the mesocolon is often a prominent feature of the disease. E. coli PWD and ED may occur independently, but they may also occur together in a single outbreak or in the same pig. PWD is endemic in many farms and its prevalence fluctuates over time and across regions. A more severe form of enteric E. coli infection may be observed at 2–3 weeks following weaning and is manifested as sudden death or severe diarrhea.
Certain E. coli, particularly those of serotype O157:H7, serogroup O26, and other non‐O157 E. coli, may be present sporadically in the intestines and feces of normal pigs and are considered as zoonotic (Zimmerman, Karriker et al. 2012).
2. Aetiology
2.1 Bacteria characteristics
The genus Escherichia is named after the German pediatrician Theodor Escherich (1857–1911). It is classified with the family Enterobacteriaceae, which consists of gram‐negative facultatively anaerobic rods. The species E. coli includes normal inhabitants of the gastrointestinal tract and strains causing a broad variety of intestinal and extraintestinal diseases in swine.
Escherichia coli are gram‐negative, peritrichously flagellated rods of variable length and with a diameter of about 1μm. Colonies on solid media reach their full size within 1day of incubation and vary from smooth to rough or mucoid. A wide range of selective media is available for growth of E. coli. Hemolysis, although not itself a significant virulence factor in enterotoxigenic E. coli (ETEC) and edema disease E. coli (EDEC), is frequently used as a marker for pathogenicity in isolates of these pathotypes producing the F4 (K88) or F18 adhesin and certain isolates producing F6 (987P). All other ETEC and an increasing number of ETEC isolates producing F4 from pigs are nonhemolytic.
Species identification may be on biochemical characteristics, although there is no differentiating biochemical test for which 100% of strains give a positive reaction. Commercially available identification kits therefore make use of up to 50 tests to achieve a high level of accuracy. Many larger diagnostic laboratories now use MALDI‐TOF mass spectrometry that generates a spectral signature that is compared with a database for the identification of the microorganism. The determination of DNA relatedness, the scientific base of discrimination between species, is restricted to research laboratories (Zimmerman, Karriker et al. 2012).
2.2 Classification
Over the decades, several classification systems have been developed to subdivide the species into types. Serotyping is an established typing system to identify pathogenic E. coli strains, and serotypes have shown good association with certain virulence traits. Complete serotyping includes determination of the surface O (somatic; polysaccharide), K (capsular or microcapsular), H (flagellar), and F (fimbrial) antigens. Unlike salmonellae, only a small percentage of E. coli isolates are typable with available antisera, since serotyping has been limited to isolates of proven or suspected pathogenicity. Presently, at least 188 O, 103 K, 56 H, and over 20 F antigens are officially recognized. A serogroup refers to strains that share only one of these antigens (e.g. serogroup O149), whereas a serotype refers to strains sharing combination of these antigens (e.g. serotype O149:H10). Although certain porcine pathogenic E. coli belong to a limited number of serotypes, serotyping is less used today for diagnostic purposes having been replaced by direct detection of genes coding for bacterial traits involved in pathogenesis of disease, called virulence factors. The term pathotype is used to identify types of E. coli on the basis of their virulence mechanism as indicated by the presence of particular virulence factor combinations, which characterize the way in which disease is caused. This system identifies broad classes or categories of pathogenic E. coli, such as ETEC; Shiga toxin‐producing E. coli (STEC), which includes the EDEC and enterohemorrhagic E. coli (EHEC); enteropathogenic E. coli (EPEC); and extraintestinal pathogenic E. coli (ExPEC) (Songer 2010). Detection of virulence factors is important for the identification of pathogenic E. coli, and the term virotype is now used to refer to the combination of these factors for a particular isolate. Table 1 summarizes important pathotypes of E. coli and their common virulence factors and serogroups as well as diseases they cause.
Table 1 Important pathotypes, adhesins, toxins, and serogroups of pathogenic E. coli in pigs (Zimmerman, Karriker et al. 2012).
Pathotype | Adhesins | Toxins | O serogroups | Diseases |
ETEC | F5(K99), | STa | O8, O9, O20, O64, O101 | Neonatal diarrhea |
F6(987P), F41 | ||||
F4(K88) | STa, STb, LT, EAST1,
| O8, O138, O141, O145, O147,
| Neonatal diarrhea | |
α‐hemolysin | O149, O157 | Diarrhea in young pigs pre-weaning | ||
F4(K88), AIDA, | STa, STb, LT, EAST1, | O8, O138, O139, O141, O147, | Post weaning diarrhea | |
unknown | α‐hemolysin | O149, O157, O?:K48 | ||
F18, AIDA | STa, STb, LT, | O8, O138, O139, O141, O147, | ||
Stx (VT), EAST1, | O149, O157 | |||
α‐hemolysin | ||||
EPEC | Eae (intimin) | O45, O103 | ||
STEC (VTEC) | F18, AIDA | Stx2e(VT2e), EAST1, α‐hemolysin | O138, O139, O141, O147 | Edema disease |
Eae (intimin) | Stx1 and/or Stx2 | O157 | None in pigs, bloody diarrhea and hemolytic uremic syndrome in humans | |
ExPEC | P, S | CNF | O6, O8, O9, O11, O15, O17, O18, O20, O45, 060, O78, O83, O93, O101, O112, O115, O116 | Colisepticemia/polyserositis |
P, S | CNF | O1, O4, O6, O18 | Urogenital infection |
Enterotoxigenic E. coli (ETEC)
ETEC is the most important pathotype in pigs and includes strains that elaborate one or several enterotoxins that induce secretory diarrhea (Fairbrother, Nadeau et al. 2005) (Songer 2010). Two major classes of enterotoxin are produced by porcine ETEC: heat‐stable toxin (ST) and heat‐labile toxin (LT). Heat‐stable toxin has been further divided into STa (also called STI, ST1, or STaP) and STb (also called STII or ST2) based on solubility in methanol and biological activity. Likewise, two subgroups of LT, LTI and LTII, have been described. The enteroaggregative heat‐stable toxin 1 (EAST1) is wide-spread among porcine ETEC. The role of this toxin in porcine diarrhea remains controversial as, alone, EAST1 does not seem capable to produce disease, but together with LT it is efficient in producing diarrhea. The E. coli enterotoxins and their activity have been reviewed in detail elsewhere (Songer 2010, Dubreuil, Isaacson et al. 2016).
ETEC must attach to the apical surface of small intestinal mucosal epithelial cells or the adjacent mucus layer in order to colonize and produce a combination of enterotoxins in the microenvironment of the glycocalyx. The level of intestinal colonization determines whether or not disease will result from infection. Bacterial cells attach by fimbrial adhesins that are hairlike appendages composed of protein subunits, extending from the bacterial cell (Figure 1). Fimbriae are classified by serologic reactivity and over 20 types have been identified. Of these, F5 (K99), F6, and F41 are generally isolated from neonatal diarrhea, F4 from both neonatal diarrhea and PWD, and F18 from PWD and ED.
Neonatal ETEC
ETEC that cause neonatal diarrhea usually produce only STa and may have one or more of the fimbriae F4, F5, F6, and F41. The prevalence of F4‐, F5‐, and F6‐fimbriated ETEC from neonatal diarrhea cases from various studies was recently reviewed, showing both temporal and geographic variations (Dubreuil et al. 2016). Of these, the F4‐ETEC most often belong to the serogroups O149, O8, O147, and O157, and the F5‐ETEC, F6‐ETEC, and F41‐ETEC to serogroups O8, O9, O64, and O101 (Songer 2010).
Post weaning ETEC
ETEC that cause diarrhea in post weaning or older suckling pigs typically produce one or more of the enterotoxins including STa, STb, LT, and EAST‐1 (Zhang, Zhao et al. 2007). These PWD strains usually have either F4 or F18 as fimbrial adhesin (Fairbrother, Nadeau et al. 2005) (Zhang, Zhao et al. 2007). However, some F4‐ and F18‐negative PWD virotypes have been identified, the most important of which are shown in Table 2. The role of these F4‐ and F18‐negative virotypes in the development of diarrhea has not yet been established. ETEC isolates of the STb or STb: EAST‐1 virotypes from weaned pigs may also produce a non‐fimbrial adhesin involved in diffuse adherence (AIDA‐I) (Ngeleka, Pritchard et al. 2003), originally detected in E. coli isolates from humans with diarrhea.
The fimbriae F4 and F18 have several variant subtypes based on antigenic differences. For F4 variants ab, ac, and ad have been described. However, almost all belong to F4ac and are often referred to simply as F4. For F18 there are two known variants, ab and ac. Though early studies associated the F18ac variant with ETEC causing PWD and F18ab with F18‐ETEC causing ED, with some exceptions, a more recent report showed that both variants may be involved in causing PWD or ED in pigs (DebRoy et al. 2009). At present in PWD, most F4‐positive isolates are O149, whereas F18 isolates are more heterogeneous and include serogroups O139, O138, O141, O147, and O157. Common serovirotypes of pathogenic ETEC from pigs with PWD are listed in Table 2.
Certain strains produce enterotoxins and Stx2e (see STEC below), as well as either of the fimbrial variants F18ab and F18ac. These strains are classified as ETEC rather than STEC, since they produce clinical PWD more than ED. Mixed infections of F18‐STEC and F4‐ ETEC may be observed. In these cases, the predominant clinical sign is often diarrhea caused by the F4‐ETEC, although histopathological evidence of ED may be present. Mixed infections of F18‐ETEC and F4‐ETEC are also observed.
ETEC may also be associated with secondary septicemia, particularly in older piglets. These isolates most frequently belong to the serovirotypes listed in Table 2.
Table 2 Common serovirotypes of pathogenic E. coli from pigs with post weaning diarrhea or edema disease (Jahanbakhsh, Smith et al. 2016).
Fimbrial adhesin | Serovirotypes |
F4 | O149:LT:STb:EAST‐1, O149:LT:STa:STb:EAST‐1, O149:LT:STb, O?:STa:EAST‐1:Stx2e |
F18 | O149:LT:STb:EAST‐1, O138:STa:STb, O138:LT:STb:EAST1:Stx2e, O139: Stx2e:(AIDA), O147:STa:STb:AIDA, O?:STa:STb, O?:STa:STb:Stx2e |
Not known | O?:STa:STb, O?:STb:EAST‐1:AIDA, O?:LT:STb:EAST‐1:AIDA, O45/O?:Eae:(EAST‐1) |
Enteropathogenic E. coli (EPEC)
Another pathotype of E. coli found in pigs with PWD is known as EPEC. EPEC was initially associated with diarrhea in children, especially in developing countries. These bacteria do not attach by fimbria; rather they have a complex secretion system that injects over 20 effector proteins into the host enterocyte, leading to intimate adherence of the bacteria to the host intestinal epithelium and to development of a characteristic “attaching and effacing” (AE) lesion (Figure 2). EPEC, which induce formation of AE lesions, are called attaching and effacing E. coli(AEEC). EPEC from different animal species may have different virulence factors, but all possess a variant of the AE factor Eae or intimin, a bacterial outer membrane protein adhesin that is responsible for intimate attachment. Hence, the presence of Eae (intimin) is indicative of an EPEC. EPEC do not possess any virulence factors of classic ETEC PWD or ED strains (Zhu, Harel et al. 1994)
Shiga toxin‐producing E. coli (STEC), edema disease E. coli (EDEC), and enterohemorrhagic E. coli (EHEC)
STEC produces one or more of a family of cytotoxins that are known as Shiga toxins (Stx) or verotoxins (VT) (Mainil 1999). The two names are used interchangeably in the literature. Many STEC without fimbriae are probably not pathogenic, but are present in the normal intestinal microbiota in pigs. In pigs the most important STEC are those that cause ED; these are known as EDEC. These strains produce the Shiga toxin variant Stx2e (VT2e) and may possess the fimbrial variant F18ab or F18ac (DebRoy, Roberts et al. 2009). Common serovirotypes of EDEC are listed in Table 2. Another subgroup of STEC, also known as EHEC, is highly pathogenic in humans (e.g. O157:H7). Most EHEC are AE, also possessing Eae and the same secretion system as EPEC. Some zoonotic EHEC are occasionally recovered, at a low rate, from pigs.
E. coli causing fatal shock
Enteric colibacillosis complicated with shock also occurs in young pigs before and after weaning. E. coli associated with this disease are usually F4‐ETEC (serogroup O149, O157, or O8) (Faubert and Drolet 1992) or Stx2e‐producing E. coli that are associated with ED.
Extraintestinal pathogenic E. coli (ExPEC)
ExPEC are a heterogeneous group of E. coli so named because their normal habitat is in the intestinal tract yet they are able to invade, cause bacteremia, and induce septicemia or localized extraintestinal infections such as meningitis or arthritis. In contrast to ETEC, EPEC, and STEC, they are not characterized by a constant group of virulence factors. Rather they possess a large number of virulence factors that vary greatly between strains. They often possess fimbrial antigens of the P, S, and F1C families that contribute to bacterial colonization (Dozois, Clément et al. 1997) as well as cytotoxins such as hemolysin and cytotoxic necrotizing factor (CNF). They usually contain one and sometimes several iron capturing systems, such as aerobactin, which permit them to survive in the blood and other tissues outside the intestines (Songer 2010). ExPEC possess lipopolysaccharides (LPS) (O‐antigen) and capsules (K‐antigens), which protect the bacteria against killing by serum complement and phagocytes.
Serogroups O6, O8, O9, O11, O15, O17, O18, O20, O45, O60, O78, O83, O93, O101, O112, O115, and O116 have most commonly been identified in isolates associated with septicemia (Songer 2010).
Coliform mastitis (CM) E. coli
CM in sows appears to be from fecal contaminants and is noncontagious. Multiple serological types of E. coli isolates from cases of mastitis may be found within a herd, between distinct glands of one sow, or even between subcomplexes within one gland. Heterogeneity of mastitis isolates has also been demonstrated by random amplified polymorphic DNA genotyping (Ramasoota, Krovacek et al. 2000). This great variety of coliform bacteria associated with CM indicates an abundant reservoir of potentially pathogenic bacteria. One study demonstrated the presence of ExPEC virulence genes in E. coli isolates from both CM cases and healthy sows, suggesting a role for other factors in CM (Gerjets, Traulsen et al. 2011).
Nonspecific urinary tract infection (UTI) E. coli
One or more of a number of bacterial species may cause nonspecific UTI, including E. coli. E. coli isolates from UTI in pigs have not been well characterized. Isolates from pyelonephritis in pigs differ from those of human uropathogenic isolates with respect to virulence profile, being rarely hemolytic and less frequently possessing P and F1C fimbriae (Krag, Hancock et al. 2009).
3. Genetics of virulence
In most E. coli diseases, virulence genes determine pathogenicity and are encoded by plasmids, bacteriophages, or pathogenicity islands (PAIs) (Songer 2010). These genes are plasmid‐encoded for enterotoxins and fimbriae or pili, phage‐encoded for Stx, and PAI‐ encoded for the AE lesion. In strains from most extraintestinal infections, however, the genes encoding for fimbriae, cytotoxins, and hemolysin are chromosomally located. In the laboratory plasmids can easily be transmitted from donor to recipient strains. However, such exchanges of genetic material do not appear to play a major role in the field as the genetic makeup of pathogenic E. coli strains is remarkably stable. This may be because a whole set of virulence factors is involved in the virulence of a particular strain, and certain recipient strains may not express transmitted plasmid‐determined functions. The clinically important development of antimicrobial resistance is an exception to this observation.
4. Epidemiology
Escherichia coli infections are widespread, occurring in both industrialized and developing countries and in temperate, subtropical, and tropical climates. Diarrhea (neonatal ETEC, PWD ETEC, and EPEC), ED due to EDEC, systemic infections due to ExPEC, CM, and UTI occur in pigs in all countries where pigs are raised commercially.
The primary habitat of E. coli in the pig is the gastrointestinal tract, and E. coli is the dominant aerobe in this habitat. E. coli populations in the pig fecal microbiota and in the farm environment are highly dynamic and show high levels of diversity (Marchant and Moreno 2013). Dominant strains change at intervals from 1day to several weeks, leading to successive waves of dominant strains (Katouli, Lund et al. 1995). It has been reported that the E. coli microbiota is highly structured, and that different compartments of the gut are preferentially colonized by distinct phylogenetic E. coli clones, though another study suggested that porcine E. coli populations might not be as structured (Schierack, Walk et al. 2007). Numbers in the large intestine average around 107 colony‐forming units (CFU)/g of contents; however, E. coli contribute less than 1% to the total bacterial count.
Escherichia coli are found in fecal‐contaminated feed, water, soil, and the environment of the pig barn. Long survival times in the environment are promoted by low temperature and sufficient moisture, among other factors. In slurry samples, a porcine E. coli O139:K82 strain remained viable for more than 11weeks (Burrows and Rankin 1970). The spread of pathogenic E. coli is presumed to mainly occur via other pigs and contaminated barn environment, but also via aerosols, contaminated feed and water, contaminated trucks that transport pigs, other vehicles, and possibly other animal species. Airborne transmission between pigs in wire cages 1.5m apart was repeatedly observed in transmission experiments with an F4‐ETEC strain (Wathes, Miller et al. 1989).
Intestinal infections caused by ETEC, EDEC, and EPEC are considered contagious. The same strain is usually found in many sick pigs and often in consecutive batches of pigs. A more recent phenomenon is the emergence in sick pigs of a more pathogenic Stx‐producing F18‐ETEC lineage demonstrating a high level of multidrug resistance, thus representing a high risk to swine production (Kusumoto, Hikoda et al. 2016). In contrast, infections caused by ExPEC and E. coli causing CM and UTI do not behave as communicable diseases. Mixed infections by more than one strain are frequent and are acquired by invasion of preexisting intestinal bacteria in the case of ExPEC and from fecal and/or environmental contamination of teats and the urethra in the case of CM and UTI.
Routine cleaning and disinfection are usually insufficient to break the cycle of infection by E. coli (Hampson, Fu et al. 1987). Under experimental conditions, however, transmission can be prevented by strict hygienic measures (Kausche, Dean et al. 1992).
There are only limited data on the susceptibility of E. coli isolates to commonly used disinfectants. According to a Danish study, E. coli fecal isolates from livestock did not appear to have developed resistance to benzalkonium chloride, H2O2, chlorhexidine, formaldehyde, or zinc chloride (Aarestrup and Hasman 2004). However, (Beier, Bischoff et al. 2005) demonstrated that reduced chlorhexidine susceptibility in virulent E. coli isolates from newborn pigs with diarrhea correlated with resistance to gentamicin and streptomycin. These findings underline the potential for transfer of this resistance and the possible impact of environmental contamination.
5. Pathogenesis
Neonatal E. coli diarrhea
Neonatal E. coli diarrhea is observed most commonly in pigs aged from 0 to 4 days and is caused by ETEC. The newborn pig, on leaving the uterus and before reaching the teats of the sow, encounters the heavily contaminated environment of the farrowing crate and the skin of the dam, resulting in ingestion of microbes from the intestinal microbiota of the sow. In conditions of poor hygiene or in a continuous‐farrowing system, buildup of pathogenic strains increases risk for an outbreak.
Pathogenesis of E. coli diarrhea
In the presence of the appropriate predisposing environmental conditions and host factors, ETEC proliferate in the intestine and cause disease by means of specific virulence factors. Risk factors for the development of various E. coli diseases in pigs are summarized in Table 3. ETEC must be able to adhere to and colonize the intestinal mucosa to permit the release of sufficient enterotoxin to cause diarrhea. Adherence to specific receptors on mucosal epithelial cells and in the adjacent mucus layer is mediated by fimbria on the bacterial surface (Figure 1).
Table 3 Risk factors for development of E. coli diseases (Zimmerman, Karriker et al. 2012).
| Etiology | Risk factors |
|
|
Diseases | E.coli pathotype+others | Host |
| Environment |
Neonatal diarrhea | F4(K88) ‐, F5(K99) ‐, F6(K987P) ‐, F41‐ETEC | ● Susceptibility to F5(K99) and F6(987P) high; decreases with age ● Susceptibility to F18 low; increases with age ● No effect of age on susceptibility to F4(K88) ● First gestation: low levels of colostral antibodies |
| Ambient temperature less than 25°C |
Septicemia | ExPEC | ● Low transfer of colostrum |
|
|
Young pig diarrhea | F4(K88) ‐ ETEC, rotavirus, coccidia, TGE virus |
|
|
|
Enteric colibacillosis complicated by shock | F4(K88) ‐ ETEC | ● Some pigs resistant to F18 due to lack of receptor |
|
|
Edema disease | F18‐STEC | ● Up to 50% of pigs may be resistant to F4 due to lack of receptor | Rapidly growing pigs | ● High protein diet ● Transportation ● Mixing of pigs ● Diet changes ● Diet elements |
Post weaning diarrhea | F4(K88) ‐, F18‐ETEC AIDA‐ETEC, EPEC, mixed E. coli pathotypes | ● Earlier weaning age ● Stress ● Loss of specific antibodies from milk |
|
|
Urogenital infection | ExPEC | ● Last period of pregnancy ● Parturition ● Trauma at coitus |
|
|
ETEC affecting neonates mostly colonize the posterior jejunum and ileum. Piglets are most susceptible to infection with F5‐ETEC and F6‐ETEC during the first several days of life and subsequently become more resistant. This susceptibility could be related to a reduction of the number of receptors present on intestinal epithelial cells with age or inhibition of colonization by preferential binding of bacteria to F6 receptors present in the mucus rather than to those on the intestinal epithelium. Not all pigs have epithelial cell receptors for F4 and are thus resistant to infection by F4‐ETEC (Van den Broeck, Cox et al. 1999). This genetic resistance is inherited in a simple Mendelian way with the allele for the receptor being dominant. A similar genetic resistance has not been observed for the other fimbriae (F5, F6, F41) of neonatal porcine ETEC.
ETEC adhering to the intestinal mucosa produce enterotoxins that change the water and electrolyte flux of the small intestine and may lead to diarrhea if the excess fluid from the small intestine is not absorbed in the large intestine. Excessive net secretion leads to dehydration, metabolic acidosis, and eventually death. Most strains of neonatal ETEC produce STa, which is active in the intestine of infant mice and young piglets of less than 2 weeks of age, but is less active in older pigs. This could be due to differences in the concentration of intestinal receptors with age. Based on the concentration and affinity of the STa receptors, the posterior jejunum appears to be the major site of hypersecretion in response to STa.
In suckling pigs, the severity of ETEC‐induced disease depends on antibody titers in the milk of the sow (Sarmiento, Dean et al. 1988). The colostrum and the milk may contain nonspecific bactericidal factors and specific antibody (mainly IgG and IgA, but also IgM) that inhibit the adherence of pathogenic E. coli to the intestine. If the dam has not been vaccinated or exposed to the pathogenic E. coli present in the environment of the piglets, specific antibodies are not present in the colostrum and the milk, and suckling piglets are susceptible to intestinal infection. Similarly, when individual piglets do not have access to colostrum, due to injury or inability to compete or due to agalactia or insufficient teats of the sow, they are more susceptible to infection.
Low ambient temperatures in the farrowing house also impact severity of disease. In pigs kept at temperatures of less than 25°C, intestinal peristaltic activity is greatly reduced, and passage of bacteria and protective antibodies through the intestine is delayed. Increased numbers of pathogenic E. coli in the intestinal tract of these pigs result in a more severe diarrhea than in pigs kept at 30°C.
Post weaning E. coli diarrhea and edema disease
Escherichia coli PWD and ED are treated in one section because they often both occur in the same age group of pigs, the causative bacteria share certain virulence factors, and some strains of E. coli can cause both diseases. There are also important differences in the two diseases.
Lactogenic passive protection decreases with aging of suckling piglets, and older unweaned piglets can be affected by E. coli diarrhea and ED as levels of protective antibody wane in maternal milk; however, loss of milk antibodies at weaning contributes significantly to susceptibility of pigs to PWD and ED (Sarmiento, Dean et al. 1988). As a result, most disease is observed post weaning.
PWD and ED are caused by strains of E. coli that possess adhesion factors enabling the bacteria to colonize the small intestine and that elaborate one or several protein exotoxins. They may occur independently, but PWD and ED may also occur in a single outbreak or in the same pig.
PWD is most commonly caused by ETEC and mediated by enterotoxins but can also be caused by EPEC that do not possess any virulence factors of classic PWD or ED strains. Common PWD and ED serovirotypes are summarized in Table 2, and virulence factors are listed in Table 1 and described in the etiology section above. PWD ETEC strains usually have either F4 or F18 variants F18ac or F18ab as fimbrial adhesins, although some F4‐ and F18‐negative virotypes exist. Most PWD F4‐positive isolates are serogroup O149, and F18 isolates are O139, O138, O141, O147, or O157. PWD ETEC are observed in pigs worldwide, and in a given geographical area, certain serovirotypes predominate (Liu, Yuan et al. 2014) (Wang, Liao et al. 2011). Out-breaks of F4‐positive E. coli disease tend to involve only one strain at any one time. Occasionally, two potential pathogens are isolated, but one usually predominates in any given outbreak. Exceptionally, in one case, multiple out-breaks in herds involving different serogroups were detected in 47% of 84 herds. Mortality is often 1.5–2% of pigs and can reach 25% if no treatment is given.
ED is caused by EDEC that colonize the small intestine and elaborate a Shiga toxin, Stx2e, which enters the bloodstream and damages vessel walls, resulting in edema in targeted tissues. Most notably, cerebral edema leads to the predominant nervous signs characteristic of the disease. Both F18ab and F18ac variants may be involved (DebRoy, Roberts et al. 2009). Most ED strains belong to serogroups O138, O139, O141, and O147. ED most often manifests as sporadic cases or small outbreaks limited to a specific age group. The case mortality rate ranges from 50 to over 90%, and the course of the disease in the herd varies from 4 to 14 days. The disease typically disappears as abruptly as it appears. Recurrence on the same site is common.
Some F18ab‐ and F18ac‐positive strains of E. coli produce both enterotoxins and Stx2e. Pigs infected by one of these strains generally exhibit clinical PWD more than ED. Outbreaks of disease may also be caused by mixed infections of both ETEC and EDEC strains. When this occurs, clinical diarrhea usually predominates even though microscopic lesions of ED may be present.
The age group primarily affected by PWD and/or ED varies based on absolute pig age and diet. There are some differences between E. coli with F4 and those with F18. Receptors for F4 are fully expressed on enterocytes of pigs from birth to adult age, rendering pigs of all ages potentially susceptible to infection. F4‐positive strains most often cause outbreaks of PWD in the very first days after weaning.
In contrast F18 receptors important in ED and some PWD are expressed age dependently. It was first reported that the F18 receptors are not yet fully expressed by piglets under about 20days of age. However, a more recent study reported that the expression of the F18 receptors in the intestines was found from 10 days of age, increasing gradually with age in the suckling period, and then maintained highly expressed from about 3 weeks of age. Hence, E. coli with F18 fimbriae do not cause diarrhea or ED in neonatal pigs and usually cause ED or PWD between 5 and 14 days after weaning or after introduction to fattening herds. Fimbrial receptors are subject to modulation by feed lectins such as constituents of leguminous plants (Kelly, Begbie et al. 1994). It is speculated that feed‐induced changes of the receptor are involved in the observed reduction in colonization by F18‐positive E. coli in the first days after weaning (Bertschinger, Stamm et al. 1993).
Older suckling pigs may acquire F18 E. coli infection from previously contaminated farrowing crates or their dam and develop disease and/or carry it into the weaner unit. The contaminated environment of the weaner unit appears to be also an important source of pathogenic E. coli strains. Intestinal infections caused by E. coli are contagious and are transmitted to other pigs by the oral route via fecal–oral transmission, contaminated immediate environment, contaminated feed and drinking water, handlers, or aerosol. The same strain is usually found in many sick pigs and often in consecutive batches of pigs (Jorsal, Aarestrup et al. 1996). These strains are usually only shed for a few days after infection, probably due to the development of immunity. Not all infected pigs develop disease; the degree of colonization determines whether disease results from infection.
Pathogenesis of PWD, ED
Colonization
When ingested in sufficient numbers, E. coli causing PWD and/or ED colonize and then proliferate rapidly to attain massive numbers to the order of 109/g of contents. For ETEC and EDEC, colonization requires attachment of fimbrial adhesins (mainly F4 and F18) to complementary receptors on the small intestinal epithelium or in the mucus coating in the mid‐jejunum to ileum. On the other hand, for EPEC, the eae adhesin (intimin) binds to its complementary receptor on the apical surface of host epithelial cells in the small and large intestines, with heaviest colonization in the duodenum and cecum.
Epithelial cell receptors for pathogenic ETEC and EDEC are not present in every pig. Certain pigs do not have receptors for F4 and are thus resistant to infection by F4‐ETEC. Receptors are prerequisite for both the development of clinical signs and immune responses with F4‐positive E. coli (Van den Broeck, Cox et al. 1999). This genetic resistance to infection is inherited in a simple Mendelian way, and the allele for the receptor is dominant. Subsequent studies have demonstrated up to eight pig phenotypes (A to H) based on susceptibility of brush borders of different pigs to adherence of isolates, producing the variants F4ab, F4ac, and F4ad. The F4 receptors per se are still unknown, and different receptor types have been proposed to play a role in the binding of F4 to enterocytes (Nguyen, Goetstouwers et al. 2013). The loci encoding porcine intestinal receptors for F4ab and F4ac are closely linked on chromosome 13 (Edfors‐Lilja, Gustafsson et al. 1995). Susceptibility of pigs to F4ab/ac ‐ETEC diarrhea has been linked to a polymorphism on MUC4 gene. However, the correlation between MUC4 polymorphism and F4 susceptibility is not completely consistent, particularly for resistance, a proportion of pigs with a MUC4‐resistant genotype showing intestinal adhesion of F4ac E. coli and/or becoming seropositive after immunization with F4 (Nguyen, Goetstouwers et al. 2013). This strongly suggests the presence of other receptor(s) for F4ac. Other genes on pig chromosome 13 have attracted scientific interest as F4ab/ac receptor, including MUC13, MUC20, and TFRC. It has been shown that pigs carrying F4ab/ac receptors had greater average daily weight gain than pigs exempt of these receptors.
Specific intestinal receptors for F18 have not yet been fully identified. However, the importance of F18 receptor in colonization of F18 E. coli and development of disease was demonstrated; the susceptibility to colonization by F18 E. coli is controlled by a dominant allele and resisted by a recessive allele (Bertschinger, Stamm et al. 1993). The receptor for F18 fimbriae is controlled in a single locus on chromosome 6 close to the locus for stress susceptibility. Pigs with at least one copy of the dominant allele for the receptor are susceptible to intestinal colonization. Susceptibility to F18 E. coli infections was reported to be dependent on the activity of the FUT1 gene, encoding the alpha (1,2)‐fucosyltransferase. The association between FUT1 M307 genotypes and adhesion phenotypes was reported to be very strong for both F18ab and F18ac. The complexity of the F18 receptor expression was recently demonstrated by differential gene expression analysis.
Other factors may also effect bacterial colonization and severity of disease. Low room temperature in the weaner rooms appears to be responsible for a more severe course of PWD (Wathes, Miller et al. 1989). This is likely because chilling reduces intestinal peristaltic activity and enhances bacterial colonization. In contrast, under experimental conditions, ED is not aggravated by cold stress (Kausche, Dean et al. 1992). Endogenous as well as orally administered proteases may reduce the receptor activity for F4 fimbriae (Mynott, Luke et al. 1996), thus reducing the severity of F4‐mediated diarrhea. Several predisposing factors, such as a weaner diets containing soya and field peas or PRRSV infection, may enhance bacterial colonization and development of the AE lesions of EPEC (Neef, McOrist et al. 1994).
Mechanisms of ETEC post weaning diarrhea
Like neonatal ETEC, post weaning ETEC adhere to the intestinal mucosa and produce enterotoxins, which change the water and electrolyte flux of the small intestine. Excessive secretion leads to dehydration, metabolic acidosis, and possibly death.
Post weaning ETEC strains produce one or more of the enterotoxins STa, STb, LT, and EAST‐1. STb is antigenically and genetically unrelated to STa and is poorly immunogenic. LT is a highly immunogenic molecule, and recent studies have shown that LT also promotes adherence of ETEC in vitro and in vivo (Fekete, Mateo et al. 2013).
EAST‐1 is commonly found in F4‐ETEC strains from pigs with diarrhea and in F18:Stx2e strains from pigs with ED. The mechanism of action of EAST‐1 is proposed to be identical to that of STa. Overall, most studies suggest that EAST1 toxin alone does not induce diarrhea in the animals tested (Dubreuil, Isaacson et al. 2016).
Mechanisms of EPEC post weaning diarrhea
EPEC use a type III secretion system (T3SS) to translocate bacterial effectors directly into the intestinal epithelial cells. Porcine EPEC attach to the intestinal mucosa and cause lesions similar to those observed for EPEC isolated from human infantile diarrhea. They attach intimately to the intestinal epithelial cell membrane by means of a bacterial outer membrane protein termed “EPEC attaching and effacing factor” (Eae) or “intimin.” Eae is the product of a chromosomal PAI in EPEC composed of over 40 genes that code for proteins involved in intimate adherence to and signal transduction within the host cell. One of these proteins is the “translocated intimin receptor” (Tir) that is injected into the host cell cytoplasm where it reappears on the host cell surface and acts as receptor for the bacterial intimin (Songer 2010). Attached EPEC efface the microvilli and sometimes invade the epithelial cells (Zhu, Harel et al. 1994). The mechanisms by which EPEC induce diarrhea are poorly understood. Effacement of microvilli and the consequent loss of absorptive surface area may lead to malabsorptive diarrhea. The rapid onset of diarrhea suggests a more active secretory mechanism that may be due to EPEC signaling activity on intracellular mediators (Songer 2010). Other possible mechanisms include an increased permeability of tight junctions between epithelial cells, a localized inflammatory response at the lesion site, or chloride secretion following polymorphonuclear (PMN) leukocyte transmigration. The clinical outcome of the infection is difficult to evaluate, as mixed infection, such as with F4‐ETEC, often occurs.
Mechanisms of edema formation by edema disease E. coli (EDEC)
ED is a Stx2e toxemia that results in severe edema in specific sites in pigs that have absorbed Stx2e from the intestine following colonization by an EDEC. Highly purified Stx2e induces a dose‐dependent disease indistinguishable from ED when administered intravenously to pigs (MAcLEOD and Gyles 1990). Colonization by EDEC develops over 3–6 days on the tips and sides of villi in the distal jejunum and ileum and is mediated by plasmid‐encoded F18ab or F18ac fimbriae. It has been reported that F18-STEC infection results in a slower colonization of the gut than F4‐ETEC, the latter showing a peak of fecal excretion at 2 days post challenge compared with 3–5 days for F18‐STEC (Verdonck, Cox et al. 2002).
The Stx2e toxin is part of the AB5 toxin family and binds to globotriaosylceramide (Gb3) or preferably to globotetraosylceramide (Gb4) receptors. The Stx2e produced by EDEC in the intestine is absorbed into the circulation and causes vascular damage in target organs. The toxin also binds to Gb4 on red blood cells. Thus, vessels are subjected to prolonged toxin exposure (Boyd, Tyrrell et al. 1993). The toxin can be detected in endothelial cells of small blood vessels of the intestine and in microvillous membranes of enterocytes at the base of the villi by immunological methods (Waddell, Lingwood et al. 1996). The Stx2e toxin does not appear to be absorbed from the intestine under normal conditions, but the addition of deoxycholate to the intestine allows absorption of Stx2e to occur, and it is possible that bile could influence absorption. Strains of EDEC may pass from the intestine to the mesenteric lymph nodes and produce Stx2e toxin there, providing another mechanism for absorption of toxin into the blood.
The most consistent injury observed in field cases, after injection of partially purified toxin (Gannon, Gyles et al. 1989) and in pigs inoculated orally with live EDEC (Kausche, Dean et al. 1992), is a degenerative angiopathy of small arteries and arterioles. The edema fluid found in various tissues is low in protein and is the result of a mild increase in vascular permeability.
A distinct type of ED is characterized by terminal bloody diarrhea and hemorrhagic lesions in the cardiac region of the stomach, the ileum, and the large intestine. (Gannon, Gyles et al. 1989) observed acute hemorrhagic gastroenteritis in some of the pigs to which a high dose of Stx2e was administered. Epithelial necrosis secondary to necrosis of small arteries and arterioles may be responsible for luminal hemorrhage.
6. Clinical signs
Clinical signs of diarrhea
Clinical signs and age most commonly affected are summarized for the various diseases caused by E. coli in Table 4. Neonatal diarrhea may first be observed 2–3 hours after birth and may affect one or a few pigs or whole litters. Gilt litters are more often affected than sow litters. Morbidity in an affected herd is extremely variable. The average is 30–40%, but may be as high as 80% in some herds. Mortality can reach 70% in affected litters. In a low proportion of pigs, the infection may be so rapid that death occurs before the development of diarrhea.
Table 4 Association of clinical signs, prevalence, and age with various clinical diseases due to E. coli (Zimmerman, Karriker et al. 2012).
Clinical disease | Age period | ||||
Newborn | Suckling | Post weaned | Grow–finish | Adult | |
Neonatal diarrhea
| x |
|
|
|
|
Severe watery diarrhea, dehydration, mortality up to 70% in affected litters | x |
|
|
|
|
Septicemia
| x |
|
|
|
|
Shock, depression, death, polyarthritis | x |
|
|
|
|
Young pig diarrhea
| x |
|
|
|
|
Low mortality, diarrhea of moderate severity, decreased weight gain |
| x |
|
|
|
Enteric colibacillosis complicated by shock
|
| x | x |
|
|
Rapid death, cyanosis of extremities, diarrhea |
| x | x |
|
|
Edema disease |
|
| x | x |
|
Sudden death, possibly paralysis, and eyelid edema, sporadic mortality up to 65% |
|
| x | x |
|
Post weaning diarrhea
|
|
| x | x |
|
Initially deaths, with severe to moderate diarrhea, decreased weight gain, mortality up to 25% in untreated animals |
|
| X | x |
|
Urogenital infection
|
|
|
|
| X |
Sporadic cystitis often after mating, pyelonephritis 2weeks postpartum |
|
|
|
| x |
Coliform mastitis |
|
|
|
| x |
First several days following farrowing, generally short duration. Clinically similar to lactational failure |
|
|
|
| X |
Diarrhea may be very mild with no evidence of dehydration or may be clear, watery, and profuse. The feces vary in color from clear to whitish or various shades of brown. In very severe outbreaks, a small proportion of affected animals may vomit. In severe cases, 30–40% of total body weight may be lost and result in signs of dehydration. Abdominal musculature may be flaccid, pigs may be sluggish with sunken eyes, and skin may be bluish gray in color and parchment‐like in texture. The loss of fluid and weight results in the exaggeration of bony prominences. These animals usually die. In more chronic or less severely affected cases, the anus and perineum may be inflamed from contact with the alkaline diarrheic fecal material. Pigs with less severe dehydration may continue to drink and, if treated appropriately, recover with only minimal long‐term effects.
Post weaning diarrhea
Diarrhea in pigs from late suckling through the post weaning period is similar to that observed in neonatal piglets but tends to be less severe. Diarrhea is seen as yellowish or grey fluid, lasting up to a week and causing dehydration and emaciation. Over several days, most of the pigs in a group may be affected, and mortality of up to 25% may be observed. Peaks of diarrhea vary between farms but are generally seen in the first 3 weeks after weaning. Severe cases have been observed at 6–8 weeks after weaning when the pigs enter the growing barns.
Edema disease
ED mostly occurs during the first few weeks after weaning, although cases may be observed through to the grower barns. The disease may be sporadic or may affect an entire herd and may be first recognized as sudden death without signs of sickness. Some affected pigsbecome inappetent, develop swelling of the eyelids and forehead, emit a peculiar squeal, and show incoordination and respiratory distress. Some of these pigs will soon become recumbent and die. There is usually no diarrhea or fever. In mild cases, subcutaneous edema is accompanied by pruritus, which disappears after recovery. In some pigs with or without dyspnea, respiration is accompanied by a snoring sound. Watery diarrhea with clots of fresh blood may appear in a few pigs at the terminal stage.
Subclinical ED may occur where pigs are clinically normal but develop vascular lesions and may have a decreased growth rate. Chronic ED occurs in a low proportion of pigs recovering from acute attacks of ED or E. coli PWD caused by strains that also produce Stx2e. This condition was called cerebrospinal angiopathy before its association with ED became apparent. For periods varying from days to several weeks after intestinal infection, growth stops, and sick pigs often show unilateral nervous disturbances such as circling movements, twisting of the head, or atrophy of limb muscles with progressive weakness. Subcutaneous edema is rare.
7. Lesions
Few specific lesions are observed. Gross lesions may include dehydration, dilation of the stomach (which may contain undigested milk curd), venous infarcts on the greater curvature of the stomach, and dilation of the small intestine with some congestion of the small intestinal wall.
Microscopically, layers of E. coli may be observed adhering in patches to the mucosal epithelial cells of the jejunum and ileum. Adhering bacteria may be found only in the crypts of Lieberkühn, or more often covering the crypts and the tips of the villi. Other lesions sometimes observed include vascular congestion in the lamina propria with hemorrhages into the intestinal lumen, increased numbers of neutrophils and macrophages in the lamina propria, and mild villous atrophy.
Post weaning diarrhea
Pigs dead from E. coli PWD are generally in good condition but severely dehydrated with sunken eyes and some cyanosis. The stomach is often distended with dry feed. The gastric fundus is variably hyperemic. The small intestine is dilated, slightly edematous, and hyperemic. The contents vary from watery to mucoid, with a characteristic smell. The mesentery is heavily congested. Contents of the large intestine most often are light greenish or yellowish and are mucoid to watery. Pigs dying late in an outbreak look emaciated and exhibit a strong smell of ammonia. There are irregularly shaped superficial ulcerations in the gastric fundus and large intestine. The feces may look yellow and pasty. If the causative strain of ETEC also produces Stx2e, lesions typical of ED (see below) are mild or absent.
Microscopic lesions in pigs with ETEC PWD are few. Bacterial layers (gram‐negative rods) may be observed in patches on the apical surface of villous epithelial cells in the ileum and, less consistently, jejunum (Sarmiento, Casey et al. 1988), although absence of this observation does not exclude ETEC infection. The mucosa and the epithelium remain normal in appearance; however, increased numbers of neutrophils may be observed in the superficial lamina propria.
Microscopically in pigs infected with EPEC, a multifocal colonization of the brush border of mature enterocytes by E. coli arranged in palisades with enterocyte degeneration and light to moderate inflammation of the lamina propria is observed, the latter being mostly in the ileum (Helie, Morin et al. 1991). Colonization is most intense in the duodenum and cecum, bacteria are sometimes observed in intracytoplasmic vacuoles in enterocytes, and colonized enterocytes swell and slough, resulting in mild to moderate villous atrophy in the small intestine. On transmission electron microscopy, bacteria are intimately attached to the cytoplasmic membrane of mature enterocytes and arranged in regular palisades, parallel to the microvilli, with effacement of adjacent microvilli. The bacterial cell wall and the apical cell membrane of the enterocyte are separated by a narrow regular gap of 10nm at the cupping pedestal, and apical dense regions are seen at attachment sites.
Edema disease
Pigs dying of ED are mostly in good condition. Edema is variable in specific sites of predilection and may be absent in some animals. Subcutaneous edema may occur and is most often in the eyelids and face (Figure 10).
Gelatinous edema that is barely detectable up to 2 cm in thickness in the submucosa of the gastric cardia and occasionally fundus is characteristic. The mesocolon is commonly edematous, and edema of the small intestinal mesentery and gallbladder is sometimes observed. The mesenteric and colic nodes may be swollen, edematous, and congested. Pericardial, pleural, and peritoneal cavities sometimes contain a slight increase of serous fluid.
The stomach is typically full of dry, fresh‐looking feed, and the small intestine is relatively empty. Some think that this is a manifestation of delayed gastric emptying, since some animals have a period of anorexia before death. Colonic contents may be diminished in amount and some pigs may be constipated. There may be varying degrees of pulmonary edema and a characteristic patchy, sublobular congestion. In some cases, this may be the only observable lesion. Cases with laryngeal edema have also been observed. A few epicardial and endocardial petechiae may occur. This lesion must not be confused with mulberry heart disease where hemorrhages are also throughout the myocardium that contains strands of fibrin.
In some pigs with ED, a form of hemorrhagic gastroenteritis occurs, which is quite different from that described with E. coli PWD. In addition to marked edema, the edematous submucosa of the cardiac region of the stomach and the mucosa of the lower small and upper large intestin show extensive hemorrhage. Watery diarrhea with clots of coagulated blood occurs shortly before death in some of these pigs.
Microscopically, patchy layers of bacteria are adherent to the distal jejuna and ileal mucosa early in the course of ED (Bertschinger and Pohlenz 1983). Contrasting with E. coli PWD, the colonization has often disappeared when pigs with ED become moribund (Bertschinger and Pohlenz 1983).
The hallmark microscopic lesion of ED is a degenerative angiopathy affecting small arteries and arterioles with associated edema of surrounding tissues. Sites of predilection are those mentioned above where edema is observed as well as in the brain. The dense arterial network in the mesocolon adjacent to the colic lymph nodes is frequently affected. Vascular lesions may be subtle, affecting only some segments of arterioles, and difficult to detect in acute cases, but are more readily apparent in surviving pigs or those affected subclinically (Kausche, Dean et al. 1992).
Acute changes include necrosis of smooth muscle cells in the tunica media evidenced by pyknotic and karyorrhectic nuclear debris and hyaline change in cytoplasm (Figure 11). In the walls of some affected vessels, fibrinoid material is deposited. Endothelial cells may also be swollen. In acute experimental cases, edema of the leptomeninges and perivascular spaces has been demonstrated. Affected vessels in the brain may be surrounded by eosinophilic, periodic acid–Schiff (PAS)‐positive droplets (Bertschinger and Pohlenz 1974) (Figure 12). In older lesions, there may be proliferation of adventitial and medial cells. Thrombosis is not usually a feature of uncomplicated naturally occurring ED.
In cases of ED where hemorrhagic gastroenteritis is observed, vessels in affected areas of the gastric, lower small intestinal, and upper colonic wall have changes strikingly similar to those observed in human hemorrhagic colitis caused by EHEC. These include swelling, vacuolation and proliferation of endothelial cells, subendothelial fibrin deposition, medial necrosis, perivascular edema, and microthrombus formation.
In pigs that have recovered from natural outbreaks or survived for several days following acute signs, there may be multifocal encephalomalacia in the brain stem together with typical lesions in the small arteries and arterioles (Kausche, Dean et al. 1992). Malacia is thought to be due to ischemia secondary to vascular injury.
8. Diagnosis
Diagnosis of diarrhea
Neonatal diarrhea caused by ETEC must be differentiated from other common infectious causes of diarrhea including Clostridium difficile and Clostridium perfringens types A and C; transmissible gastroenteritis virus (TGEV); porcine epidemic diarrhea virus (PEDV); rotavirus groups A, B, and C; and PRRSV. In suckling pigs 5 days and older, Isospora suis must also be considered. It may be helpful to determine fecal pH as ETEC produces alkaline feces, whereas malabsorptive diarrheas produced by TGEV, PEDV, and rotaviruses produce acidic feces.
Diagnosis of neonatal ETEC is based on clinical signs, microscopic lesions, and the presence of small gram‐negative rod‐shaped bacteria colonizing the small intestinal mucosa. Colonization can be visualized in formalin‐fixed, paraffin‐embedded tissues by routine histopathology, or E. coli organisms can be definitively identified and visualized using immunohistochemistry or in frozen sections using indirect immunofluorescence.
This diagnosis is strengthened by the isolation from rectal swabs or intestines of E. coli possessing appropriate virulence factors. Criteria used to identify causative E. coli in diarrhea in pigs are summarized in Table 5.
Table 5 Criteria used to identify causative E. coli in diarrhea (Zimmerman, Karriker et al. 2012).
Pathogenic E. coli identified | |||||||
ETEC | |||||||
Criterion | F4(K88) | F18 | F5(K99), F6(987P), F41 | AIDA | F18‐STEC | EPEC | ExPEC |
Hemolytic colonies | All (not discriminatory) | None | All | None | Some | ||
OK serotyping | Most (some non‐ETEC identified) | Not known | Most | Few | Some | ||
F adhesin serotyping | All | Not reliable | Most (not reliable) | Not done | Not reliable | Not done | |
Virotyping | All | Some |
Swabs or samples of intestinal contents should be inoculated onto blood and MacConkey agar or other media, which are selective for Enterobacteriaceae and allow differentiation of lactose fermenting from lactose‐non‐fermenting gram‐negative enteric bacilli. Use of transport medium such as alginate swabs or Stuart’s medium should be considered if isolation could not be done within 24 hours.
Morphology and lactose fermentation on MacConkey agar are a first indication of potential E. coli. To identify the species as E. coli, it is essential to determine the capacity of colonies to transform indole, since 99% of E. coli strains are indole positive. Identification can be completed by the citrate assay (E. coli are not able to use citrate as the only carbon source) and by the methyl red assay.
A diagnostic laboratory can use OK typing sera (serotyping) against serogroups most prevalent in its geographical region to obtain a rapid presumptive diagnosis. Common O serogroups associated with disease in pigs are shown . Complete O and H serotyping can only be carried out in a few reference laboratories.
The use of serotyping for primary identification of porcine pathogenic E. coli is less relevant today because of the presence of non‐typable (NT) pathogenic strains, not all strains of a given serotype are pathogenic, the need of large number of reference antisera, and the growing availability of efficient genetic methods to identify virulence factors. Virotyping, or determination of the virulence factors, is thus a more definitive way of identifying pathogenic E. coli.
The presence of enterotoxins and cytotoxins may be detected by tests for biological activity, which are no longer commonly used in routine diagnosis. Antigen ELISA tests have been developed for the detection of Stx‐producing cultures or for direct detection of the Stx‐producing bacteria in the feces and may be used more widely in diagnostic laboratories.
Slide agglutination, with or without latex particles, is a simple and easy method to determine the presence of fimbrial adhesins of ETEC, which are expressed in culture media, except F18, which is rarely expressed in vitro. This method is commonly used for the identification of F4‐ETEC. Antigen ELISA is also used to determine the presence of adhesins. However, bacteria must be grown on the appropriate media for detection of F5 and F41, which are only well expressed in culture media low in glucose or alanine. Other fimbriae, such as F1 and F6, undergo phase variation and may be very poorly expressed after several passages in the laboratory.
Currently, genotypic analysis is more commonly used to define the virotypes involved in an infection. Techniques include colony or DNA hybridization and polymerase chain reaction (PCR) for the detection of genes encoding for virulence factors such as toxins and adhesins (Harel, Lapointe et al. 1991). PCR may also be used to detect pathogenic E. coli in situ in formalin‐fixed, paraffin‐embedded tissues.
Diagnosis of PWD, ED
In enteric post weaning E. coli infections, a presumptive diagnosis is made based on clinical presentation of diarrhea early after weaning (or sometimes later as previously described), marked dehydration, and low to moderate mortality. Mild diarrhea may be the only clinical presentation in less severe cases. The gross lesions, including the characteristic smell, are also helpful. Differentials should include other common infectious causes of non‐bloody diarrhea in weaned pigs including rotaviruses, TGEV, salmonellosis, PEDV, and, in older weaned pigs, proliferative enteropathy.
Likewise in ED, a presumptive diagnosis is made based on the sudden appearance of neurologic disease in thriving pigs in the first weeks after weaning. Partial ataxia or a staggering gait is the most consistent sign, and subcutaneous edema in the palpebrae and over the frontal bones is also a cardinal sign when present. Sudden death without clinical signs has been observed in some cases. Characteristic lesions of edema in gastric mucosa and mesentery are helpful when present, but may be absent in a significant number of cases, especially when severe diarrhea has preceded ED. Differentials should include other common causes of nervous signs in weaned pigs including pseudorabies, teschoviral encephalitis, Streptococcus suis or Haemophilus parasuis induced meningitis, and water deprivation/salt intoxication. ED should be included in the differential diagnostic when sudden death is observed in the first weeks after the weaning.
Definitive diagnosis of ETEC or EPEC PWD and ED is based on the presence of typical lesions and culture of the causative E. coli along with confirmation of appropriate virulence factors. Criteria used to identify causative E. coli in diarrhea in pigs are summarized in Table 5. Serotyping continues to be used today in some laboratories, but virotyping (identification of virulence factors) should be performed to confirm the case. Colonization can be visualized in formalin‐fixed, paraffin‐embedded tissues by routine histopathology, or E. coli organisms can be definitively identified and visualized using immunohistochemistry or in frozen sections using indirect immunofluorescence. However, absence of observed colonization does not exclude ETEC or EDEC. Methods for culture and serovirotyping are discussed previously in the section on diagnosis of neonatal ETEC.
Culture of the small intestine in ETEC and small intestine and colon in EPEC and ED usually yields pure or nearly pure cultures of hemolytic (ED and ETEC) or nonhemolytic (EPEC) E. coli. However, in ED bacterial numbers may have declined in more protracted cases (Bertschinger and Pohlenz 1983); therefore, a negative bacteriologic result does not exclude the diagnosis of ED. Because all F18 and most F4 E. coli causing ED and ETEC PWD are hemolytic, the presence of hemolytic colonies is often used as a rapid means for confirming a presumptive diagnosis of these conditions. However, this method would exclude EPEC and an increasing number of F4‐ETEC that produce nonhemolytic colonies on blood agar. This may be an important consideration when putting into place prevention strategies, such as vaccination. In addition, in cases of diarrhea with mixed infections of hemolytic and nonhemolytic ETEC, the assumption that the presence of hemolytic colonies indicated that F4‐ or F18‐ETEC was the only causative agent may result in the nonhemolytic ETEC remaining undetected. Likewise, in cases of diarrhea mixed with subclinical ED, this assumption would result in the ED remaining undetected. These are important considerations in light of the increasing prevalence of mixed pathogenic E. coli infections associated with cases of diarrhea, particularly later in infection or in groups with a more endemic presentation.
In cases of subacute or chronic ED or of ED in adults, culture is of little value since causative EDEC are typically no longer a dominant strain in the intestines. Subacute or chronic ED is diagnosed by lesions, especially by the demonstration of subacute to chronic arteriopathy and possibly lesions of focal encephalomalacia. Diagnosis of ED in adult pigs may require additional effort, often requiring postmortem examination and histopathology of more than one pig. Death of adults is occasionally caused by cerebral hemorrhage (stroke) from rupture of severely damaged arterioles, with an apparent affinity for the basal ganglia, especially the corpus striatum.
Table 6 Differential diagnosis of the main agents of neonatal diarrhoea (Luppi 2017).
Disease/Etiological Agent | Age | Diarrhoea | Gross Lesions | Lethality | Laboratory diagnostic methods |
Colibacillosis E. coli (ETEC) | Most commonly from 0 to 4 days | Yellowish, grey or slightly pink alkaline pH | Distension, congestion of small intestine. Stomach full of curdled milk | Can reach 70% | Culture/isolation. Typing of isolates usually by PCR Histopathology |
Clostridiosis C. perfrigens type C | PA: 1 days A: 3 days SA: 7 days C:10–14 days | PA: watery yellowish bloody A: brown bloody SA: watery grey/yellow C: yellow/grey | Jejunum and ileum mostly involved. Haemorrhagic enteritis Bloody ascitis | 100% in PA and A forms | Culture/isolation. Typing/toxin identification. Histopathology |
Clostridiosis C. perfrigens type A | Generally diarrhoea is observed within 48 hours of birth | Mucoid, pink without blood | Jejunum and ileum mostly involved Pasty content Presence of necrotic membrane | Generally low if not complicated | Culture/isolation. Typing/toxin identification. Histopathology |
Clostridiosis Clostridium difficile | In the first week of life | Pasty and yellow | Mesocolon oedema. Typhlocolitis with focal erosions | Variable. Up to 50% | Culture/isolation. Toxin identification |
Coronavirus PEDV TGEV | All | Watery yellow/white/ grey Watery yellow, white, grey, greenish; acid pH | Empty stomach. Small intestine was thinned and congested | Differs between strains and between naïve and endemic infected herds. Very high (80–100%) in suckling piglets belonging to naïve infected herds | PCR Histopathology Viral isolation |
Rotaviral enteritis Rotavirus | From 1 to 5 weeks | Watery, sometime pasty. Acid pH | Small intestine was thinned. Milk in the stomach | Low (in endemic infected herds) <20% | PCR Histopathology Viral isolation |
Coccidiosis Isospora suis | Not before 5 days. More frequent around 14 days | Yellow and pasty. Alkaline pH | Small intestine. Enteritis with fibrino-necrotic membrane | Very low or not observed | Microscopic evaluation after flotation |
PA per-acute, A acute, SA sub-acute, C chronic
Table 7 Differential diagnosis of the main agents of post-weaning diarrhoea (Luppi 2017).
Disease/ Etiological Agent | Age | Diarrhoea | Gross Lesions | Lethality | Laboratory diagnostic methods |
Colibacillosis E. coli (ETEC, EPEC) | Most commonly post-weaning until 45–50 days | Yellowish, grey or slightly pink alkaline pH | Distension, congestion of small intestine. Gastritis and stomach full of feed | Can reach 25% | Culture/isolation. Typing of isolates usually by PCR. Histopathology |
Swine dysentery Brachyspira hyodysenteriae | Frequent in the growing-fattening periods | Muco-haemorrhagic | Muco-haemorrhagic and fibrino-necrotic typhlocolitis | Variable, usually low | Culture/isolation. Typing by PCR. Histopathology |
Salmonellosis (Salmonella typhimurium) | Mostly in the growing-fattening periods | Yellowish, greenish, muco-haemorrhagic | Necrotic lesions yellowish membrane (small and large intestine); Prominent Payer patches | Low | Culture/isolation |
PED and TGE Coronavirus PEDV TGEV | All | Watery yellow/white/ grey Watery yellow, white, grey, greenish; acid pH | Empty stomach. Small intestine was thinned and congested | Can be high; less severe than in neonates | PCR Histopathology Viral isolation |
Rotaviral enteritis Rotavirus | From 1 to 5 weeks | Watery, sometime pasty. Acid pH | Small intestine was thinned. | Low, <20% | PCR Histopathology Viral isolation |
Proliferative enteropathy Lawsonia intracellularis | Post-weaning | A: haemorrhagic C: greenish | Ileitis | Low | PCR Histopathology |
A acute, C chronic
9. Control and prevention of disease
Prevention and control of diarrhea
Treatment with antimicrobials at the earliest sign of diarrhea may be on an individual or litter basis, by mouth or parenteral injection. It is important to confirm the diagnosis of E. coli infection by culture and to perform antimicrobial sensitivity tests because antimicrobial sensitivity varies greatly among E. coli isolates. In vitro resistance of E. coli isolates to a wide range of antimicrobial agents has dramatically increased over the last several years. Commonly used antimicrobials are ampicillin, apramycin, ceftiofur, gentamicin, neomycin, spectinomycin, furazolidone, and potentiated sulfa drugs. An alternative approach to the antibiotic treatment of enteric E. coli infection is the use of bacteriophages, an approach that has been successful experimentally (Jamalludeen, Johnson et al. 2009) but has not been extensively applied in the field.
Fluid therapy, consisting of electrolyte replacement solutions containing glucose given orally, is useful for the treatment of dehydration and acidosis. Drugs that inhibit the secretory effects of enterotoxin, such as chlorpromazine and berberine sulfate, may be useful for the treatment of diarrhea, although many of these drugs have undesirable side effects. The use of such anti secretory drugs as bencetimide and loperamide, alone or in combination with antibacterial agents, has also been suggested (Solis et al. 1993).
The principles of control of E. coli neonatal diarrhea revolve around hygiene and management strategies to reduce the buildup of the causative E. coli and increasing immunity in sows and piglets. A program for prevention of enteric E. coli infection should be aimed at reduction of numbers of pathogenic E. coli in the environment by good hygiene, maintenance of suitable environmental conditions, and provision of a plentiful supply of colostrum at birth and a high level of immunity. Strategies commonly used for the control of enteric E. coli infections are summarized in Table 6.
One of the most important factors in prevention of enteric E. coli infection and septicemia is the maintenance of piglets at an adequate environmental temperature, free of drafts, and on a low‐heat‐conducting floor. This is particularly true for piglets of below average weight, which lose heat more rapidly because they have a greater skin surface area per unit body weight.
Stringent biosecurity should be used to control the introduction of different E. coli virotypes or other infectious agents into the herd. Animals in the herd will have little immunity to E. coli fimbrial antigens with which they have not had contact.
Table 8 Strategies commonly used for the control of enteric E. coli infections (Zimmerman, Karriker et al. 2012).
Strategies that result in: | ||
Reduced number of pathogenic E. coli | Increased resistance of animals to infection | |
Preweaning diarrhea | ●Warmth ● Hygiene ● Gate and floor design ● Quarantine ● All‐in/all‐out ● Farrowing | ● Maternal vaccination – F4(K88), F5(K99), – F6(987P), F41 ● Pig hyperimmune – γ‐globulin |
Post weaning diarrhea and edema disease | ● Increase weaning age ● Warmth ● Diet – Highly digestible – Milk‐based protein – Restricted feed intake ● Hygiene ● Water additive – Organic acids ● Feed supplements – Organic acids – ZnO – Spray‐dried plasma – Probiotics ● Antimicrobial peptides | ● Live oral nontoxigenic F4(K88) and F18 E. coli vaccines ● Oral powdered egg yolk from F4(K88) ‐ and F18‐ immunized hens ● Stx2e toxoid vaccine (edema disease) ● Selection of F4(K88) ‐ and F18‐resistant animals |
Good hygiene in the farrowing area leads to a reduction in the numbers of E. coli presented to the piglet to a level that it is able to control through its own defense mechanisms. Farrowing crates should be thoroughly cleaned and disinfected between litters, and farrowing rooms should be managed as all‐in/all‐out with thorough disinfection between farrowing groups. Sow fecal material should be minimized in farrowing crates by sizing crates to sow size that minimize area of fecal contamination and by utilizing perforated flooring that allows fecal material to drop through. Litters farrowed onto such floors have a noticeably lower incidence of diarrhea than those on solid concrete floors.
A dry, warm environment reduces the moisture available for survival and growth of E. coli. This is largely affected by ventilation rates, although if room temperature is too high, sows tend to try and spread water over their lying area to cool themselves, thereby defeating other hygienic procedures. The sow should be at a temperature of approximately 22°C, necessitating a warmer creep area for the piglets. It is important to ensure that younger piglets are maintained at a constant temperature of 30–34°C to prevent chilling, which predisposes to neonatal colibacillosis.
Maternal vaccination has been one of the most effective ways of preventing neonatal ETEC diarrhea in piglets. One of the earliest vaccination techniques consisted of taking the small intestinal contents (or scour) from a piglet with diarrhea, culturing it in milk, and feeding the culture to pregnant sows, usually about a month before parturition. This technique is effective, conferring an immunity lasting throughout the suckling period, and is still used, particularly in the United States (Zimmerman, Karriker et al. 2012).
Commonly used commercially available vaccines are given parenterally and may be killed whole cell bacterins or purified fimbrial vaccines, with or without LT toxoid. Both types of vaccines appear to work equally well. Bacterins usually contain strains representing the most important serogroups and producing the fimbrial antigens F4, F5, F6, and F41 (Songer 2010). Two doses are usually given parenterally at 3 or 4 weeks apart, the second generally administered 2–3 weeks prior to parturition. Most vaccines comprise antigens for other pathogens, such as for C. perfringens, and rotavirus. Some vaccines may include antigens for the different F4ab, F4ac, and F4ad variants. However, it is not clear that these vaccines would be more efficient than others including only F4ac since most cases are due to F4ac‐ ETEC and immunologic cross‐reactivity between the three variants is reported. One sow vaccine including also bacterins of F18ab and F18ac E. coli has been recently approved in several European countries. In cases where vaccination is ineffective, it is important to identify the serotype of the isolated causative strain for possible inclusion in an autogenous bacterin. Further characterization of these isolates may identify new or variant fimbrial adhesins important in the pathogenesis of ETEC diarrhea.
Table 9 Classification of antibacterial drugs frequently used in the treatment of enteric colibacillosis according to pharmacokinetics and pharmacodynamics indices (Luppi 2017).
Drugs | Bacterial effect | PK/PD parameters |
Fluoroquinolones (enrofloxacin) | Concentration dependent | Cmax/MIC and AUC/MIC |
Cephalosporins (ceftiofur) | Time dependent | T > MIC |
Sulphonamides + Diaminopyrimidines (trimethoprim + sulfamethoxazole) | Time dependent | T > MIC |
Aminoglycosides (neomycin) | Concentration dependent | Cmax/MIC or AUC/MIC |
Aminocyclitols (spectinomycin) |
| T > MIC |
Polymyxins (colistin) | Concentration dependent | AUC/MIC |
Table 10 Main antimicrobials used in swine for the treatment of enteric colibacillosis: routes of administration, dosages and main pharmacokinetic properties (Luppi 2017).
Antimicrobial class/ compounds | Administration and dosage (mg/kg body weight) | Pharmacokinetic Properties | Administration in enteric colibacillosis | |||
Injection | In water | In feed | ||||
Trimethoprim/ sulfonamide | 15 (2.5 + 12.5) | 30 (5 + 25) | 15 (2.5 + 12.5) | Rapidly absorbed from intestine, well distributed in tissues; crosses uninflamed blood–brain barrier | IM and orally | |
Amoxicillin | 7 | 20 | 15–20 | IM and orally | ||
Amoxicillin + Clavulanic acid | 7 + 1,75 | IM | ||||
Ceftiofur | 3 | Poorly absorbed from intestine, relatively poorly distributed in tissues; crosses only inflamed blood–brain barrier | IM | |||
Cefquinome | 1–2 | IM | ||||
Enrofloxacin | 2.5 | Well absorbed and distributed in tissues | IM | |||
Neomycin | 11 | 11 | Poorly absorbed from intestine, relatively poorly distributed in tissues | Orally | ||
Apramycin | 7.5–12.5 | 4–8 | Poorly absorbed from intestine, relatively poorly distributed in tissues | Orally | ||
Spectinomycin | 10–50 | 1.1–2.2 | Poorly absorbed from intestine, relatively poorly distributed in tissues | Orally | ||
Colistin sulphate | 100,000 IU/Kg body weight | 100,000 IU/Kg body weigh | Not absorbed from intestine | Orally |
Prevention and control of PWD, ED
Treatment
In post weaning colibacillosis, treatment with antimicrobials and electrolytes should be administered. Sick pigs must be treated parenterally as they eat and drink very little. Subsequently the antimicrobial may be given in the water or feed or as metaphylaxis treatment. Attractive rehydration fluid should be offered to counteract dehydration and acidosis or injected intraperitoneally if the pig is anorectic. Such fluids may contain glucose, glycine, citric acid, and potassium dihydrogen phosphate in an isotonic solution. Uptake should be equal to the loss (i.e. up to 25% of the body weight).
Control of bacterial proliferation therapeutically is much more effective in E. coli PWD than in ED, because in ED Stx2e has already been absorbed into the circulation and been bound to receptors when clinical signs become visible. In general, pigs showing neurological signs have a poor prognosis. The development of bacterial resistance against a wide range of antimicrobial drugs makes the efficacy of antimicrobial therapeutics uncertain. Antimicrobial susceptibility testing is indispensable for selection of effective drugs. Antimicrobials that reach therapeutic concentrations in the intestinal lumen, such as amoxicillin/clavulanic acid, fluoroquinolones, cephalosporins, apramycin, ceftiofur, neomycin, or trimethoprim, must be selected. However, antimicrobials identified as “critically important in human medicine” such as fluoroquinolones and cephalosporins of third and fourth generations, should be used only as a last resort.
For groups of pigs during outbreaks of ED, withholding the feed is thought to impair colonization and is a valuable measure still recommended to prevent new clinical cases. In‐feed or water medication, selected by sensitivity testing of isolated EDEC, administered a few days before the onset of ED can reduce the outcome of the outbreak (Zimmerman, Karriker et al. 2012).
Preventive husbandry
Nurseries should be managed as all‐in/all‐out facilities and should be thoroughly cleaned of organic matter and disinfected prior to use. Water lines and water systems should likewise be disinfected using shock chlorination or a similar chemical process. Management of the weanling pig should minimize environmental and other forms of stress such as unnecessary mixing of litters, chilling, transportation, and assignment to new pens. Recently weaned pigs should be housed in a draft‐free environment at a constant temperature of about 29.5°C.
Passive and active immunoprophylaxis
Various strategies involving passive immunity for the prevention of PWD and ED have been used with varying success. An improved weight gain and lower frequency of diarrhea were observed in early weaned (10 days of age) pigs fed a spray‐dried porcine plasma‐based diet, partly due to the presence of specific anti‐ETEC antibodies (Owusu-Asiedu, Baidoo et al. 2002). Similarly, spray-dried porcine plasma had an inhibitory effect on E. coli enterotoxemia that lasted only as long as the plasma was fed. However, the potential link between spray‐dried porcine plasma products and PEDV transmission in North America led to a ban, or voluntary removal, of these products in several North American and European areas. Passive immune protection against colonization with F4‐ and F18‐positive E. coli may be attained by feeding eggs from vaccinated hens or IgY from egg yolk, although effectiveness is not always observed in challenge studies (Li, Wang et al. 2015). Antiserum produced by injecting a horse with Stx2e toxoid was effective in protecting pigs from ED in two Danish herds with this disease (Zimmerman, Karriker et al. 2012).
Several commercial vaccines are now available for the prevention of E. coli PWD and ED. Vaccination against ETEC in pigs has been recently reviewed (Nguyen, Goetstouwers et al. 2013). Injectable vaccines, such as those administered to sows for the prevention of neonatal diarrhea, stimulate mostly systemic rather than mucosal immunity, giving rise to circulating antibodies, which do not reach intestinal bacteria in high enough levels to be very effective (Van den Broeck, Cox et al. 1999). Such vaccines may even suppress the mucosal immune response upon subsequent oral infection with a pathogenic E. coli. Though systemic induction of serum IgA by parenteral vaccines may result in the presence of secretory IgA in the intestinal tract, the latter would be present only as long as the systemic response persists, as no antigen‐specific IgA antibody‐secreting cells are present in the gut‐associated lymphoid tissue (GALT) (Melkebeek, Goddeeris et al. 2013). A new vaccine comprising different E. coli bacterins was recently approved in some European countries for vaccination of sows and gilts for the passive immunization of piglets in order to prevent neonatal diarrhea and to reduce mortality and clinical signs due to PWD (up to 21 days of age) and to ED (up to 28 days of age).
Live attenuated and wild‐type avirulent E. coli vaccines have been shown to be an effective approach to prevent and control F4 and F18 pathogenic E. coli. Live nonpathogenic E. coli vaccine strains carrying fimbrial adhesins may be administered to weaned piglets in the drinking water or to unweaned piglets by oral drenching at least one week prior to the expected onset of diarrhea. Oral administration of a live nonpathogenic F4 E. coli vaccine to 17‐ to 18‐day‐old pigs immediately following weaning induced a significant protection after challenge with a F4‐ETEC strain administered at 7 and 21 days after vaccination and resulted in reduction of clinical signs of PWD, ileal colonization and fecal shedding of F4‐ETEC, and normal growth rates after challenge (Fairbrother, Nadeau et al. 2017). Live nonpathogenic F4 E. coli vaccines are commercially available (Europe and North America), and a bivalent live vaccine comprising a F4 nonpathogenic E. coli strain and a F18 nonpathogenic E. coli strain has been recently approved in Europe and Canada for immunization of pigs against F4‐ and F18‐positive E. coli PWD.
Another approach is the oral administration of purified F4 and/or F18 fimbriae as a vaccine for the control of outbreaks of E. coli‐associated diarrhea in weaned pigs reviewed by (Melkebeek, Goddeeris et al. 2013). The use of such experimental subunit vaccines, with or without the mucosal adjuvant CT, results in a specific intestinal mucosal immune response and a significant reduction in fecal excretion of the pathogenic F4 E. coli. Contrary to F4, oral delivery of purified F18 could not induce a protective response against pathogenic F18 E. coli challenge infection.
Several approaches for the control of ED have been investigated. A genetically modified Stx2e toxoid was found to prevent overt and subclinical ED when vaccinated pigs were challenged with EDEC. In two Danish herds with persistent ED problems, vaccination with a Stx2e toxoid almost totally eliminated mortality due to ED (Johansen, Andresen et al. 1997). A commercial injectable vaccine based on genetically modified recombinant Stx2e that showed reduction of mortality due to ED is now licensed in Europe and Canada.
As with ETEC PWD, live avirulent F18‐positive E. coli strains have been orally administered to pigs at least 1week prior to the expected onset of ED. Commercial oral live vaccines comprising an avirulent F18 E. coli are available in North America for immunization of recently weaned pigs against ED, but no efficacy studies are yet published. The abovementioned bivalent F4/F18 live vaccine comprising a F4 and a F18 nonpathogenic strain has been recently licensed in Canada for immunization of pigs against F4‐PWD, F18‐PWD, and F18‐ED.
Antimicrobial prophylaxis
At present preventive feed medication with antibiotics is widely practiced in most countries despite serious drawbacks such as nonacceptance by the consumer, impaired buildup of immunity, and selection of resistant bacteria. Resistance is often induced within days or a few weeks. Isolates from E. coli PWD and ED show the highest rate of resistance within porcine E. coli. In addition to the classes of antimicrobials mentioned above for parenteral therapy, aminoglycosides and colistin are widely used for chemoprophylaxis. However, colistin resistance in pigs has been increasingly reported worldwide in the last several years. In addition, since the first description of the plasmid‐mediated colistin resistance mcr‐1 gene in food animals, food, and humans in China in November 2015, the number of reports in animal sources rapidly increased. Recently, the European Antimicrobial Advice Ad Hoc Expert Group of the European Medicines Agency recommended that colistin should be classified as “critically important antimicrobial” (category 2), reserved for situations when there is no affective alternative (Zimmerman, Karriker et al. 2012).
Dietary preventive measures
A number of nutritional strategies appear promising as alternative means of maintaining performance and controlling PWD in weaned pigs without using in‐feed antimicrobials and have been reviewed elsewhere.
Restriction of feed intake, high fiber diets, and ad libitum feeding of fiber have been reported as effective deterrents to the development of ED and PWD (Bertschinger, Eggenberger et al. 1979). The nutritive value of the feed may be reduced by increasing fiber content to 15–20% and reducing crude protein and digestible energy to one‐half of the normal values. The addition of fiber to normal diets or feeding high‐quality alfalfa coupled with restriction of daily feed intake may be beneficial. A low protein diet with amino acid supplementation may decrease production of toxic protein metabolites and reduce PWD. Animal source proteins seem to provide protection against PWD. Addition of dairy products to feed delayed the occurrence of PWD and reduced mortality, possibly due to greater digestibility or stimulation of higher feed intake (Zimmerman, Karriker et al. 2012).
A lower mortality due to E. coli enterotoxemia and improved weight gains were reported after introduction of rations with a reduced acid‐binding capacity. A similar effect is ascribed to organic acids. Organic acids contribute to the maintenance of an acidic gastrointestinal tract, which may control potentially pathogenic bacteria. However, mortality due to ED was not reduced by the inclusion of a mixture of organic and inorganic acids in the feed. This result is not surprising in view of the highly regulated pH close to the mucosal surface.
Zinc oxide may offer an alternative to antimicrobials. Feeds with contents between 2400 and 3000ppm of zinc reduce diarrhea and mortality and improve growth. The prevention of disease in piglets improves growth and feed conversion efficiency. Positive response at 1500ppm has been reported using growth rate as an indicator. The protective effects of zinc oxide may be due not to antibacterial activity but to a protection of intestinal cells from ETEC infection by inhibition of bacterial adhesion and internalization and modulation of cytokine gene expression. However, care must be taken to avoid overdose or feed pigs for a too long period since toxicity has been reported. Environmental considerations should be included in discussions of zinc oxide at such high levels. Recently, different studies suggest that therapeutic doses of zinc coselect for antibiotic resistance development in weaned pigs, though further research is needed to confirm the link between the use of zinc and antimicrobial resistance.
Several successful dietary supplements have been reported. Oral administration of immunostimulatory beta‐glucans from the cell wall of yeasts reduces the susceptibility of weaned piglets to F4‐ETEC infection. Exogenous as well as endogenous proteases lower the activity of intestinal F4 receptors. Bromelain, a protease from pineapple stems, administered orally to pigs reduced the binding of F4‐ETEC to brush borders in a dose‐dependent manner. Wide ranges of antimicrobial peptides, which are components of the host defense system, have been identified, which may potentially replace antibiotics. Other alternatives include clay minerals, essential oils, and recombinant enzymes, although most of these compounds produce inconsistent results and they are rarely as effective as antibiotics (Zimmerman, Karriker et al. 2012).
Addition of colicin E1, a member of a class of bacteriocins produced by and effective against E. coli, in the diet of young pigs decreased the incidence and severity of PWD caused by F18‐ETEC and improved the growth performance of the piglets. Prebiotics selectively stimulate the proliferation of potentially beneficial microorganisms in the gastrointestinal tract. For example, administration of a heat‐killed and dried Enterococcus faecalis strain significantly reduced the incidence of clinical signs due to STEC.
Some promising results have been obtained using probiotics, potentially beneficial microorganisms. Feeding of a diet supplemented with a Lactobacillus sobrius strain isolated from the pig intestine resulted in a significant decrease in ETEC numbers and increased daily weight gain in weaned pigs challenged with an F4‐ETEC. Lactobacillus plantarum, given to piglets in early life, improved performance and effectively prevented the diarrhea in young piglets induced by F4‐ETEC challenge by improving function of the intestinal barrier by protecting intestinal morphology and intestinal permeability. The administration of Pediococcus acidilactici or Saccharomyces cerevisiae boulardii was effective in reducing F4‐ETEC attachment to the ileal mucosa, and at least the presence of P. acidilactici resulted in the modulation of the expression of intestinal inflammatory cytokines, in pigs challenged with F4‐ETEC. On the other hand, others reported no efficacy of feeding of Lactobacillus spp., Enterococcus faecium, and Bacillus cereus strain “toyoi” to experimentally and/or naturally infected pigs (Zimmerman, Karriker et al. 2012).
Feeding of a diet supplemented with fermented soy beans (especially Rhizopus‐fermented soybean, but also Bacillus‐fermented soybean) reduced the excretion of ETEC and the incidence, severity, and duration of diarrhea in weaned pigs.
Breeding of resistant pigs
Augmentation of the presence of both the F18 and F4 resistance loci in the pig population through breeding is an attractive approach to prevent PWD and ED. However, it will be important to avoid co‐selection of unwanted traits closely linked with loci coding for the F18 and F4 receptors. It cannot be predicted if additional types of adhesive fimbriae or new variants of known types will emerge, which could bind to yet unidentified receptors. Availability of techniques for large‐scale selection of resistant animals remains an important challenge.
A few genetic markers are used by some genetic and breeding companies to increase the proportion of F4‐and F18‐resistant pigs. A PCR‐RFLP test detecting FUT1 M307 polymorphism, correlated with the gene controlling expression of the E. coli F18 receptor, could be a simple and inexpensive method for large‐scale selection of animals (Frydendahl, Jensen et al. 2003).
The transmembrane mucins MUC13 and MUC4 provide potential markers for selection of ETEC F4ab/ac resistant animals, although it is probable that other receptors are involved. However, (Rasschaert, Verdonck et al. 2007) calculated the sensitivity and specificity for the in vitro villous adhesion test, with MUC4 genotyping as the gold standard, as 100 and 24%, respectively. Low specificity was evidenced by a high proportion of pigs identified as genetically resistant showing adhesion of F4ac E. coli on the intestine. On the other hand, the immunity aspect should be considered if breeding for resistance becomes available for F4‐ETEC, as resistant sows (F4 receptor‐negative sows) do not develop and transfer F4‐specific antibodies in their colostrum. Thus, heterozygous piglets are not passively protected from development of neonatal diarrhea due to these strains.
LIST OF PRODUCTS RECOMMENDED BY VEMEDIM
Group of product | Product name | Dosage | Image |
Rehydration | 100g/ 200 liters of drinking water.
| ||
30mL per 25kg bodyweight | |||
Antibiotic | Ceftiofen | 1 mL/20-33 kg b.w/day, in 3 consecutive days | |
Vime-SOC | 1 ml/10 kg b.w/day. | ||
Supportive products | Atropin | 1ml for 5 - 7.5 kg of b.w. | |
2-5ml/ animal
| |||
0.5-2.5mg/kg |
10. References
Aarestrup, F. M. and H. Hasman (2004). "Susceptibility of different bacterial species isolated from food animals to copper sulphate, zinc chloride and antimicrobial substances used for disinfection." Veterinary microbiology 100(1-2): 83-89.
Beier, R., et al. (2005). "Chlorhexidine susceptibility, virulence factors, and antibiotic resistance of beta-hemolytic Escherichia coli isolated from neonatal swine with diarrhea." Bulletin of Environmental Contamination & Toxicology 75(5).
Bertschinger, H., et al. (1979). "Evaluation of low nutrient, high fibre diets for the prevention of porcine Escherichia coli enterotoxaemia." Veterinary microbiology 3(4): 281-290.
Bertschinger, H. and J. Pohlenz (1974). "Cerebrospinale Angiopathie bei Ferkeln mit experimenteller Coli-Enterotoxamie." Schweizer Archiv fur Tierheilkunde.
Bertschinger, H. and J. Pohlenz (1983). "Bacterial colonization and morphology of the intestine in porcine Escherichia coli enterotoxemia (edema disease)." Veterinary pathology 20(1): 99-110.
Bertschinger, H., et al. (1993). "Inheritance of resistance to oedema disease in the pig: experiments with an Escherichia coli strain expressing fimbriae 107." Veterinary microbiology 35(1-2): 79-89.
Boyd, B., et al. (1993). "Alteration of the glycolipid binding specificity of the pig edema toxin from globotetraosyl to globotriaosyl ceramide alters in vivo tissue targetting and results in a verotoxin 1-like disease in pigs." The Journal of experimental medicine 177(6): 1745-1753.
Burrows, M. and J. Rankin (1970). "A further examination of the survival of pathogenic bacteria in cattle slurry." British Veterinary Journal 126.
DebRoy, C., et al. (2009). "Comparison of genotypes of Escherichia coli strains carrying F18ab and F18ac fimbriae from pigs." Journal of veterinary diagnostic investigation 21(3): 359-364.
Dozois, C. M., et al. (1997). "Expression of P, S, and F1C adhesins by cytotoxic necrotizing factor 1-producing Escherichia coli from septicemic and diarrheic pigs." FEMS Microbiology Letters 152(2): 307-312.
Dubreuil, J. D., et al. (2016). "Animal enterotoxigenic Escherichia coli." EcoSal Plus 7(1).
Edfors‐Lilja, I., et al. (1995). "The porcine intestinal receptor for Escherichia coli K88ab, K88ac: regional localization on chromosome 13 and influence of IgG response to the K88 antigen." Animal Genetics 26(4): 237-242.
Fairbrother, J. M., et al. (2017). "Immunogenicity and protective efficacy of a single-dose live non-pathogenic Escherichia coli oral vaccine against F4-positive enterotoxigenic Escherichia coli challenge in pigs." Vaccine 35(2): 353-360.
Fairbrother, J. M., et al. (2005). "Escherichia coli in postweaning diarrhea in pigs: an update on bacterial types, pathogenesis, and prevention strategies." Animal health research reviews 6(1): 17-39.
Faubert, C. and R. Drolet (1992). "Hemorrhagic gastroenteritis caused by Escherichia coli in piglets: clinical, pathological and microbiological findings." The Canadian Veterinary Journal 33(4): 251.
Fekete, P. Z., et al. (2013). "Both enzymatic and non-enzymatic properties of heat-labile enterotoxin are responsible for LT-enhanced adherence of enterotoxigenic Escherichia coli to porcine IPEC-J2 cells." Veterinary microbiology 164(3-4): 330-335.
Frydendahl, K., et al. (2003). "Association between the porcine Escherichia coli F18 receptor genotype and phenotype and susceptibility to colonisation and postweaning diarrhoea caused by E. coli O138: F18." Veterinary microbiology 93(1): 39-51.
Gannon, V., et al. (1989). "Effects of Escherichia coli Shiga-like toxins (verotoxins) in pigs." Canadian Journal of Veterinary Research 53(3): 306.
Gerjets, I., et al. (2011). "Comparison of virulence gene profiles of Escherichia coli isolates from sows with coliform mastitis and healthy sows." Veterinary microbiology 152(3-4): 361-367.
Hampson, D., et al. (1987). "Investigation of the source of haemolytic Escherichia coli infecting weaned pigs." Epidemiology & Infection 99(1): 149-153.
Harel, J., et al. (1991). "Detection of genes for fimbrial antigens and enterotoxins associated with Escherichia coli serogroups isolated from pigs with diarrhea." Journal of Clinical Microbiology 29(4): 745-752.
Helie, P., et al. (1991). "Experimental infection of newborn pigs with an attaching and effacing Escherichia coli O45: K" E65" strain." Infection and immunity 59(3): 814-821.
Jahanbakhsh, S., et al. (2016). "Dynamics of extended-spectrum cephalosporin resistance in pathogenic Escherichia coli isolated from diseased pigs in Quebec, Canada." International journal of antimicrobial agents 48(2): 194-202.
Jamalludeen, N., et al. (2009). "Evaluation of bacteriophages for prevention and treatment of diarrhea due to experimental enterotoxigenic Escherichia coli O149 infection of pigs." Veterinary microbiology 136(1-2): 135-141.
Johansen, M., et al. (1997). "Prevention of edema disease in pigs by vaccination with verotoxin 2e toxoid." Canadian journal of veterinary research 61(4): 280.
Jorsal, S., et al. (1996). Oedema disease in Danish pig herds, transmission by trade of breeding animals. Proc 14th IPVS Congress, Bologna, Italy.
Katouli, M., et al. (1995). "Phenotypic characterization of intestinal Escherichia coli of pigs during suckling, postweaning, and fattening periods." Applied and environmental microbiology 61(2): 778-783.
Kausche, F., et al. (1992). "An experimental model for subclinical edema disease (Escherichia coli enterotoxemia) manifest as vascular necrosis in pigs." American journal of veterinary research 53(3): 281-287.
Kelly, D., et al. (1994). "Nutritional influences on interactions between bacteria and the small intestinal mucosa." Nutrition Research Reviews 7(1): 233-257.
Krag, L., et al. (2009). "Genotypic and phenotypic characterisation of Escherichia coli strains associated with porcine pyelonephritis." Veterinary microbiology 134(3-4): 318-326.
Kusumoto, M., et al. (2016). "Emergence of a multidrug-resistant Shiga toxin-producing enterotoxigenic Escherichia coli lineage in diseased swine in Japan." Journal of clinical microbiology 54(4): 1074-1081.
Li, X., et al. (2015). "Chicken egg yolk antibodies (IgY) as non-antibiotic production enhancers for use in swine production: a review." Journal of animal science and biotechnology 6(1): 1-10.
Liu, W., et al. (2014). "Frequency of virulence factors in Escherichia coli isolated from suckling pigs with diarrhoea in China." The veterinary journal 199(2): 286-289.
Luppi, A. (2017). "Swine enteric colibacillosis: diagnosis, therapy and antimicrobial resistance." Porcine health management 3(1): 1-18.
Macleod, D. L. and C. L. Gyles (1990). "Purification and characterization of an Escherichia coli Shiga-like toxin II variant." Infection and Immunity 58(5): 1232-1239.
Mainil, J. (1999). "Shiga/verocytotoxins and Shiga/verotoxigenic Escherichia coli in animals." Veterinary research 30(2-3): 235-257.
Marchant, M. and M. A. Moreno (2013). "Dynamics and diversity of Escherichia coli in animals and system management of the manure on a commercial farrow-to-finish pig farm." Applied and environmental microbiology 79(3): 853-859.
Melkebeek, V., et al. (2013). "ETEC vaccination in pigs." Veterinary immunology and immunopathology 152(1-2): 37-42.
Mynott, T., et al. (1996). "Oral administration of protease inhibits enterotoxigenic Escherichia coli receptor activity in piglet small intestine." Gut 38(1): 28-32.
Neef, N., et al. (1994). "Development of large intestinal attaching and effacing lesions in pigs in association with the feeding of a particular diet." Infection and Immunity 62(10): 4325-4332.
Ngeleka, M., et al. (2003). "Isolation and association of Escherichia coli AIDA-I/STb, rather than EAST1 pathotype, with diarrhea in piglets and antibiotic sensitivity of isolates." Journal of Veterinary Diagnostic Investigation 15(3): 242-252.
Nguyen, V., et al. (2013). "Differentiation of F4 receptor profiles in pigs based on their mucin 4 polymorphism, responsiveness to oral F4 immunization and in vitro binding of F4 to villi." Veterinary immunology and immunopathology 152(1-2): 93-100.
Owusu-Asiedu, A., et al. (2002). "Response of early-weaned pigs to spray-dried porcine or animal plasma-based diets supplemented with egg-yolk antibodies against enterotoxigenic Escherichia coli." Journal of Animal Science 80(11): 2895-2903.
Ramasoota, P., et al. (2000). "Identification of Escherichia coli recovered from milk of sows with coliform mastitis by random amplified polymorphic DNA (RAPD) using standardized reagents." Acta Veterinaria Scandinavica 41(3): 249-259.
Rasschaert, K., et al. (2007). "Screening of pigs resistant to F4 enterotoxigenic Escherichia coli (ETEC) infection." Veterinary microbiology 123(1-3): 249-253.
Sarmiento, J., et al. (1988). "Postweaning diarrhea in swine: experimental model of enterotoxigenic Escherichia coli infection." American journal of veterinary research 49(7): 1154-1159.
Sarmiento, J., et al. (1988). "Effects of weaning on diarrhea caused by enterotoxigenic Escherichia coli in three-week-old pigs." American journal of veterinary research 49(12): 2030-2033.
Schierack, P., et al. (2007). "Composition of intestinal Enterobacteriaceae populations of healthy domestic pigs." Microbiology 153(11): 3830-3837.
Songer, J. G. (2010). "Histotoxic clostridia." Pathogenesis of bacterial infections in animals 4: 203-209.
Van den Broeck, W., et al. (1999). "Receptor-dependent immune responses in pigs after oral immunization with F4 fimbriae." Infection and immunity 67(2): 520-526.
Verdonck, F., et al. (2002). "Different kinetic of antibody responses following infection of newly weaned pigs with an F4 enterotoxigenic Escherichia coli strain or an F18 verotoxigenic Escherichia coli strain." Vaccine 20(23-24): 2995-3004.
Waddell, T. E., et al. (1996). "Interaction of verotoxin 2e with pig intestine." Infection and immunity 64(5): 1714-1719.
Wang, X.-M., et al. (2011). "Serotypes, virulence genes, and antimicrobial susceptibility of Escherichia coli isolates from pigs." Foodborne pathogens and disease 8(6): 687-692.
Wathes, C., et al. (1989). "Cold stress and post-weaning diarrhoea in piglets inoculated orally or by aerosol." Animal Science 49(3): 483-496.
Zhang, W., et al. (2007). "Prevalence of virulence genes in Escherichia coli strains recently isolated from young pigs with diarrhea in the US." Veterinary microbiology 123(1-3): 145-152.
Zhu, C., et al. (1994). "Virulence properties and attaching-effacing activity of Escherichia coli O45 from swine postweaning diarrhea." Infection and Immunity 62(10): 4153-4159.
Zimmerman, J. J., et al. (2012). Diseases of swine, John Wiley & Sons.
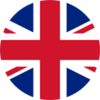