Nguyen Pham Thao Nhi, Tran Duy Thanh, Pham Trang Thanh Nguyen,
Nguyen Khanh Thuan, Nguyen Phuc Khanh, Nguyen Thanh Lam*
1.Introduction
Leptospirosis is a cause of reproductive loss in breeding herds and has been reported in swine from all parts of the world. Endemic infection in a herd of swine may produce little evidence of clinical disease, but when it is first introduced into a susceptible breeding herd, or during periods of waning herd immunity, it can cause very appreciable losses through infertility, abortion, and the full‐term birth of dead pigs or weak pigs of reduced viability.
Leptospires persist in the kidneys and female and male genital tracts of carrier swine and are excreted in urine and genital fluids. Survival outside the host is favored by warm moist conditions. Transmission is by direct or indirect contact with a carrier pig or other animal. Interruption of transmission from infected pigs or other hosts to susceptible pigs is the critical factor in control (Zimmerman, Karriker et al. 2012).
2.History
The modern history of leptospirosis began in 1886 when Adolph Weil described a particular type of jaundice accompanied by splenomegaly, renal dysfunction, conjunctivitis, and skin rashes. It was subsequently named Weil’s disease. However, it is apparent that leptospirosis had existed for millennia. Although it is difficult to draw firm conclusions from records before the advent of modern medical and scientific literature, it seems clear that at least some of the early disease outbreaks described in ancient texts were leptospirosis. For example, ancient Chinese texts carry accounts of “rice field jaundice”, while in Japan syndromes clearly recognizable today as leptospirosis were termed “autumn fever” or “seven-day fever”. In Europe, Australia and elsewhere, associations were recognized between febrile illness and particular occupations, giving rise to syndromes such as “cane-cutter’s disease”, “swine-herd’s disease”, and “Schlammfieber (mud fever)”, well before the common etiology was recognized and identified. For a more detailed description of the early accounts of what were almost certainly large-scale outbreaks of leptospirosis.
3. Geographic distribution
Leptospirosis has worldwide distribution due to the large spectrum of mammalian hosts that harbor and excrete the agent from their renal tubules (Ko, Goarant et al. 2009). According to (Guerrant, Walker et al. 2011) although leptospirosis is one of the wide spread zoonoses in the world, it is more common in the tropical regions, because of the longer survival of leptospires in the environment and frequent exposure of animals and human to contaminated environments (Guerrant, Walker et al. 2011). China, Southeast Asia, Africa, South and Central America have immense areas where the disease is endemic. Serovars of L. interohaemorrhagiae, L. canicola, L. pomona, L. hardjo and L. gripotyphosa occur in all continents except Antarctica and outbreaks in animals and humans have been reported following natural disaster such as flooding and hurricane.
4. Aetiology
4.1 Morphology of Leptospira
Leptospira have a Gram-negative-like cell envelope consisting of a cytoplasmic and outer membrane. However, the peptidoglycan layer is associated with the cytoplasmic rather than the outer membrane, an arrangement that is unique to spirochetes. The two flagella of Leptospira extend from the cytoplasmic membrane at the ends of the bacterium into the periplasmic space and are necessary for the motility of Leptospira (Mohammed, Nozha et al. 2011).
Leptospires are corkscrew-shaped bacteria, which differ from other spirochaetes by the presence of end hooks. They belong to the order of Spirochaetales, family Leptospiraceae, genus Leptospira about 0.1 µm in diameter by 6–20 µm in length. Leptospires are mobile, their bodies are small diameter requiring the use of dark field microscopy or phase contrast for observation. These bacteria are aerobic, do not resist drought or hypertonicity, however, they support alkalinization to pH 7.8. Leptospira species are also divided serologically through the cross reaction of cell antigens using the cross agglutinin absorption test (CAAT); over 200 serovars have been described for the genus. Leptospires have distinctive hooked ends (Figure 2A & 2B). Two periplasmic flagella with polar insertions are located in the periplasmic space and are responsible for motility; the flaA and flaB proteins constitute the flagellar sheath and core respectively. Electron microscopy showed a flaB mutant to be deficient in endoflagella and non-motile. Leptospires have a typical double membrane structure in which the cytoplasmic membrane and peptidoglycan cell wall are closely associated and are overlaid by an outer membrane. Within the outer membrane, the LPS constitutes the main antigen for Leptospira. It is structurally and immunologically similar to LPS from Gram negative organisms. Their visualization is achieved after metal impregnation (silver staining) or after artificial thickening by immunoperoxidase or immunofluorescence.
Leptospires are obligate aerobes with an optimum growth temperature of 28–30°C. They grow in simple media enriched with vitamins B1 and B12, long-chain fatty acids, and ammonium salts. Long-chain fatty acids are utilized as the sole carbon source and are metabolized by β-oxidation. Growth of leptospires is often slow on primary isolation, and cultures have to be retained for about 13 weeks before being discarded. Agar may be added at low concentrations (0.1– 0.2%). In such semisolid media, growth reaches a maximum density in a discrete zone beneath the surface of the medium, which becomes increasingly turbid as incubation proceeds. This growth is related to the optimum oxygen tension and is known as a Dinger’s ring or disk. Leptospiral cultures are maintained by repeated subculture or by storage in semisolid agar containing hemoglobin. Long-term storage in liquid nitrogen also yields good results and is the preferred method of storage for maintaining virulence. Molecular diagnostic methods are increasingly being used for clinical diagnosis in endemic areas because of their sensitivity and specificity. PCR amplification techniques should help to characterize any Leptospira DNA sequences present. Especially in the early stage of an outbreak, it can be extremely valuable to characterize further any diagnostic DNA sequences that have been amplified in order to confirm the amplification as being definitively derived from Leptospira and not due to an anomalous amplification. This can be done by hybridization, restriction endonuclease digestion or DNA sequencing. Each of these approaches, however, requires the use of additional reagents and equipment and thus adds to the complexity of the diagnostic process (Mohammed, Nozha et al. 2011).
4.1 Morphology of Leptospira
Figure 2. High-resolution scanning electron micrograph of Leptospira interrogans serovar copenhageni (Mohammed, Nozha et al. 2011).
4.2 Classification
The genus Leptospira includes both saprophytic and pathogenic species. The pathogenic family consists of 14 pathogenic species: Leptospira alexanderi, Leptospira alstonii, Leptospira borgpetersenii, Leptospira broomii, Leptospira inadai, Leptospira interrogans, Leptospira fainei, Leptospira kirschneri, Leptospira kmetyi, Leptospira licerasiae, Leptospira noguchii, Leptospira santarosai, Leptospira weilii, and Leptospira wolffii, with more than 260 serovars. There are seven saprophytic species. There are differences in the global distribution of some of the Leptospira species: L. interrogans, L. borgpetersenii, and L. kirschneri have a worldwide distribution, whereas L. noguchii and L. santarosai are found mainly in North and South America, while L. weilii is found mainly in China and Eastern Asia. Strains that cause disease in pigs are found mainly in the L. interrogans and L. borgpetersenii species (Zimmerman, Karriker et al. 2012).
4.3 Pathogenicity
The mechanisms of leptospiral pathogenesis are poorly understood; however, recently, significant progress has been made. The whole-genome sequences of the pathogenic L. interrogans serovars Lai and Copenhageni, and L. borgpetersenii serovar Hardjo, as well as two strains of the free-living L. biflexa are currently available. The leptospiral genome is made up of approximately 3.9–4.6 Mbp, depending on the strain and species, and is composed of two circular chromosomes. A third circular replicon, designated as p74, is found in L. biflexa, but not in pathogenic strains; however, 13 housekeeping genes found in p74 are present in chromosome I of the pathogenic strains. p74 has been implicated in the survival of L. biflexa, because mutations in the housekeeping genes affected the viability of this saprophytic strain. The L. borgpetersenii genome is 700 kb smaller than that of L. interrogans, which is indicative of genome reduction. Some genes necessary for environment sensing as well as metabolite transport and utilization are lost in this strain, limiting L. borgpetersenii survival outside of host animals. L. interrogans, on the other hand, can persist in aquatic environments until it encounters a mammalian host. A better understanding of the pathogenic pathways and the virulence factors involved is currently under investigation with the availability of genome sequences, genetic tools and animal models that can be utilized for different studies (Evangelista and Coburn 2010).
5. Epidemiology
5.1 Susceptible hosts
Maintenance hosts: An animal infected with a host-adapted serovar of the organism is a maintenance or reservoir host. Each serovar is adapted to a particular maintenance host, although they may cause disease in any mammalian species. A serovar behaves differently within its maintenance host species and incidental or accidental hosts (Radostits, Gay et al. 2006). The disease is maintained in nature by chronic infection of the renal tubules of these maintenance hosts (Yadeta, Bashahun et al. 2016).
Maintenance host is characterized by a high susceptibility to infection, endemic transmission within the host species, relatively low pathogenicity for its host, tendency to cause chronic rather than acute disease, producing insidious economic loss through reproductive losses, persistence of the serovar in the kidney and sometimes the genital tract, low antibody response to infection and low efficacy of vaccination in prevention of infection. Examples of this relationship are serovar bratislava in swine and serovar hardjo bovis in cattle (Radostits, Gay et al. 2006). The primary reservoir hosts for most Leptospira serovars are wild mammals, particularly rodents and reservoir hosts among domestic animals includes cattle, dogs, sheep and pigs and they may act as carriers for several months (temporary carrier) while rodents usually remain carrier throughout their life (permanent carrier). Rodents are therefore considered as the major reservoir of infection.
Accidental (Incidental) hosts: Exposure of susceptible animals to non–host-adapted serovars results in accidental or incidental disease. Incidental host is characterized by relatively low susceptibility to infection but high pathogenicity for the host, a tendency to cause acute and severe rather than chronic disease. An example of this relationship is infection by serovar Pomona in cattle which is pig adapted serovar (Radostits, Gay et al. 2006). Humans are incidental hosts for Leptospira species (Yadeta, Bashahun et al. 2016).
5.2 Transmission
The main sources of infection of the disease are urine of infected or carrier animals, contaminated surface water, mud, feed, soil, aborted fetuses and uterine discharges. From these sources the organism enters the body via mucous membranes of the eyes, nose, vagina, or abraded skin. The modes of transmission of leptospirosis are often categorized as direct or indirect depending upon the immediate source of infection. When the immediate source of infection is animal tissue, body fluids, urine, transplacental, or venereal the transmission is termed as direct. When the immediate source of infection is an environment contaminated with urine of carrier animals, the transmission is termed as indirect. Has reported sexual transmission of Leptospira by mating in rats, pigs and dogs. Infected milk and semen of an infected bull may contain leptospirae, so transmission through milk and natural breeding or artificial insemination can occur but it is uncommon. Transmission to human is through direct or indirect contact of mucous membranes or abraded skin with urine from infected animals or contaminated freshwater surfaces including mud or water in lakes, rivers and streams. Ingestion or inhalation of contaminated water or aerosols may also result in infection. Transmission between humans is very rare and it occurs through blood transfusion, organ transplantation, breast feeding and sexual intercourse (Yadeta, Bashahun et al. 2016).
6. Pathogenesis
The most important route of natural infection has not been determined. It is thought to be via the mucous membranes of the eye, mouth, or nose. Infection via the vaginal route is also possible, and transmission through milk from an infected dam has been demonstrated experimentally. A period of bacteremia, which may last for a week, begins 1 or 2 days after infection. During this period leptospires can be isolated from most organs of the body and also from cerebrospinal fluid.
This primary bacteremic phase ends with the appearance of circulating antibodies, which are detectable usually after 5–10days. A secondary bacteremic period (after 15–26 days) has been reported in experimental Hardjo infection. Anti-leptospiral agglutinins appear at detectable levels in the blood at approximately 5–10 days after infection and reach maximum levels at around 3 weeks. Peak titers vary considerably (1:1,000 to 1:100,000 in the MAT), and these may be maintained for up to 3 weeks, after which a subsequent gradual decline occurs. Low titers may be detectable for several years in many animals.
Following the period of leptospiremia, leptospires localize in the proximal renal tubules where they multiply and are voided in the urine. The duration and intensity of urinary shedding varies from pig to pig and with the infecting serovar. In the case of Pomona infection, the intensity of excretion is highest during the first month of shedding, when more than a million leptospires may be present in each milliliter of urine; urine shedding is very constant during this period (Zimmerman, Karriker et al. 2012).
A variable period of intermittent, low‐intensity leptospiruria then ensues, and this may last for up to 2 years in some cases. Leptospires also localize in the uterus of pregnant sows, and abortion, production of stillborn pigs, and neonatal disease frequently result from intrauterine infections occurring in the last half of the gestation period. Abortions and stillbirths usually occur 1–4 weeks following infection of the gilt or sow (Hanson and Tripathy 1986), by which time most sows have developed detectable antibody titers. Since pig fetuses are capable of producing antibodies during the latter stages of gestation, some stillborn piglets will have detectable titers.
The pathogenesis of reproductive disease is poorly understood, but some authors believe that transplacental infection, occurring during the very limited period of maternal leptospiremia, is the sole cause. While this may be true for systemic infections such as Pomona, the low antibody titers detected in sows aborting Bratislava infected fetuses has led to the hypothesis that infection occurs as a result of waning uterine immunity being unable to prevent transplacental infection by leptospires present in the genital tract. Horizontal transmission tolittermates not infected during the period of maternal leptospiremia may also occur. Once the placental barrier is breached, septicemia results in large numbers of leptospires in all fetal tissues (Zimmerman, Karriker et al. 2012).
An additional feature seen in Bratislava infection but not reported for the other swine leptospiral infections is the persistence of leptospires in the oviduct and uterus of nonpregnant sows and in the seminal vesicles, bulboure-thral glands, prostate, and testes of boars.
7. Clinical signs and pathology
7.1 Clinical signs
The vast majority of swine leptospiral infections are subclinical. Two groups of pigs are most likely to experience clinical infections: the young piglet and the pregnant sow.
Acute leptospirosis
This phase usually coincides with the period of bacteremia. In experimental infections, many pigs exhibit transient anorexia, pyrexia, and listlessness at this time. However, the mild nature of these signs means that in natural infections, especially in endemically infected herds where perhaps only one or two animals may be affected, this phase of infection usually goes unrecognized.
There have been a few reports of jaundice and hemoglobinuria in naturally occurring outbreaks, particularly in cases of infection in piglets under 3 months of age by icterohemorrhagiae. A high proportion of these undergo spontaneous recovery within a week of when clinical signs develop. The small number of such reports suggests that this more severe form of disease is rare (Zimmerman, Karriker et al. 2012).
Chronic leptospirosis
Abortions, stillbirths, the birth of weak piglets of reduced viability, and reduced litter size are primary signs of chronic leptospirosis, particularly Pomona infection. It is this aspect of leptospirosis that can cause considerable economic loss. Information as to the importance of leptospirosis as a cause of abortion in national swine herds is not available. If it were, it would vary from country to country depending on prevalence as well as epidemiologic and management factors, including the implementation of control measures. From the limited and dated information available, it would appear that even in countries where vaccination has been widely practiced, leptospirosis is a common cause of swine abortion. In Ontario, for example, 6% of swine abortions were attributed to Pomona infection. Endemic Tarassovi infection was considered to be the cause of a 3% abortion rate in herds in Poland. (Fearnley, Wakeley et al. 2008) found 4 of 24 fetal diagnostic submissions from the United Kingdom to be PCR positive. Acute outbreaks can still give rise to severe losses; (Saraví, Molinari et al. 1989) described an outbreak in a herd in which 19% of pregnant sows aborted, while the proportion of stillborn per litter rose from 8% prior to the outbreak to 28% during the outbreak. Differences in strain pathogenicity also contribute to different prevalences of clinical abortion in infected herds.
A very high prevalence of serovars belonging to the Australia serogroup in aborted pig litters has been observed in part of the United Kingdom. Similar strains have also been recovered from aborted piglets in the United States. Reported fetal Bratislava infection following abortions in 16% of sows in a herd in Ontario. Reported FAT positive prevalences ranging from 5 to 23% in diagnostic submissions in Ireland. Published experimental evaluations of the significance of such microbiological findings are not available. There has, however, been an absence of significant isolations of other abortifacient agents from these cases, and the farrowing rate and the number of live piglets born/sow improves significantly following either Bratislava vaccination or the use of an antibiotic medication program (Zimmerman, Karriker et al. 2012).
Following abortions due to Pomona, there does not appear to be any subsequent limitation on reproductive performance, even in pigs that remain infected for long periods (Mitchell, Robertson et al. 1966, Kemenes and Süveges 1976).
Infertility is a feature of Bratislava infection (Hathaway 1981, Van Til and Dohoo 1991). Split‐herd trials, carried out using a Bratislava bacterin, have demonstrated significant improvements in sow fertility (Frantz, Hanson et al. 1989).
7.2 Pathology
Leptospirosis is characterized by the development of vasculitis, endothelial damage, and inflammatory infiltrates composed of monocytic cells, plasma cells, histiocytes, and neutrophils. On gross examination, petechial hemorrhages are common and may be extensive, and organs are often discolored due to the degree of icterus. The histopathology is most marked in the liver, kidneys, heart, and lungs, but other organs may also be affected according to the severity of the individual infection. The overall structure of the liver is not significantly disrupted, but there may be intrahepatic cholestasis. Hypertrophy and hyperplasia of Kupffer cells is evident, and erythrophagocytosis has been reported. In the kidneys, interstitial nephritis is the major finding, accompanied by an intense cellular infiltration composed of neutrophils and monocytes. Leptospires can be seen within the renal tubules. By electron microscopy, the tubular cell brush borders are denuded, the tubular basement membrane is thickened, and tubular cells exhibit mitochondrial depletion. In addition, minor changes are seen in the glomeruli, suggesting an anatomical basis for proteinuria in leptospirosis.
Pathological findings in the heart include interstitial myocarditis with infiltration of predominantly lymphocytes and plasma cells, petechial hemorrhages (particularly in the epicardium), mononuclear infiltration in the epicardium, pericardial effusions, and coronary arteritis. In the lungs, pulmonary congestion and hemorrhage are common, and infiltration of alveolar spaces by monocytes and neutrophils occurs. Hyaline membrane formation may occur. Leptospires may be seen within endothelial cells in interalveolar septa, and attached to capillary endothelial cells.
In skeletal muscles, particularly of the leg, focal necrosis of isolated muscle fibers occurs, with infiltration of histiocytes, neutrophils, and plasma cell. This evidence of myositis correlates with the intense myalgia reported by some patients. In brain, perivascular cuffing is observed.
8. Diagnosis
8.1 Differential diagnosis
The principal differential diagnosis is porcine reproductive and respiratory syndrome. Other infectious causes of pregnancy loss include brucellosis, parvovirus, and porcine circovirus.
8.2 Laboratory diagnosis
Direct evidence:
Dark-field microscopy: Microscopy of blood is of value only during the first few days of the acute illness. Dark field microscopy (DFM) examination of body fluids such as blood, urine and CSF has been used. Approximately 104 leptospires/ml are necessary for one cell per field to be visible by DFM. It is a simple method, but may not be positive if there are few bacteria in the sample. Double centrifugation of the sample at low speed to separate the cellular elements, and then at high speed, help concentrate the leptospires (Mythri 2015).
Phase contrast microscopy: Phase contrast microscopy is useful for visualizing leptospires in the laboratory, but, because of its technical limitations in thick suspensions and its optical characteristics, it has no practical purpose whenever DFM is available.
Silver deposition techniques: The standard stains for spirochaetes have long been silver deposition methods. These depend on the ability of the method to selectively coat the surfaces of the leptospires with a deposit of silver. They require a large number of intact organisms to be present; artefacts are a major problem smears made on slides can be stained with Fontana stain. Leptospires can be stained in formalin fixed tissue sections by the Dobell method for blocks, or Warthin-Starry, Faine, or Dieterle methods for cut sections. (Mythri 2015).
Immunofluorescence: Immunofluorescence (IF) is useful in examination of urine, other body fluids, and tissues that have been frozen or are not amenable to silver staining. IF is often preferable to silver staining because it is easier to see leptospires, especially in small numbers, and the serovars or serogroups can be determined presumptively. When a combination of antisera labelled with different fluorochromes is used, more than one serological type of leptospires can be identified in the same preparation. One disadvantage is the need for special fluorescent microscopy equipment; another is that specially prepared labelled antisera are required. This is a fast and reliable test where facilities are available. The antiserum used must be carefully evaluated for cross-reactivity with other bacteria and for specificity for the particular serovar(s) of interest. IF has not been used widely for primary diagnostic tests.
Immunohistochemistry: This can be achieved without the need for special equipment by using enzymatic or metallic labels on the secondary antibody. Phosphatase, peroxidase, or metallic gold-labelled antibody can be used in a variety of formats to stain leptospires in clinical specimens. This technique has the advantage of being useful with formalin-fixed tissue. Using modern methods of antigen retrieval in immunohistochemistry, leptospiral antigens can be detected and stored for considerable periods of time, making this technique particularly useful for retrospective studies (Mythri 2015).
Nucleic acid probes and hybridization: Leptospira specific sequences are isolated, cleaved and labelled with a reporter molecule. The labelled DNA in the single stranded (ss) form is then hybridized to ss DNA in the sample. If the nucleotide sequences in the nucleic acid probe are complementary to those in the sample, hybridization occurs and results in the form of nucleic acid hybridization which are monitored by autoradiography in the case of probes labelled with radioactive material, or calorimetrically with non-radioactive material.
Polymerase chain reaction (PCR): PCR can rapidly confirm the diagnosis in the early phase of the disease, when bacteria may be present and before antibody titres are at detectable levels. PCR can be applied to blood, urine, cerebrospinal fluid and tissue samples. Ophthalmological complications of leptospirosis can be diagnosed directly and positively by PCR on aqueous humor. PCR requires dedicated laboratory space and also highly skilled personnel. It may give false positive results in the presence of minute amounts of extraneous DNA that may contaminate working areas. It may also give false negative results if inhibitors are present in the clinical materials that are being examined. The main limitations of PCR-based methods are the need of special equipment, the relatively high cost of the reagents and the absence of automated and standardised procedures allowing the testing of large sets of samples and the inability of most PCR assays to identify the infecting serovar.
Culture of Leptospira: Leptospires can be recovered from blood or CSF obtained from patients during the septicaemic stage of illness or from urine during the immune stage. For routine use, Fletcher semisolid medium or Ellinghausen-McCullough-Johnson-Harris (EMJH) semisolid medium is recommended. Blood: Blood should be cultured in the first 10 days of the illness and before antibiotics are given. Cultures are incubated at 28 to 300 C in the dark for 6 wks or longer. All culture tubes are examined with DFM at 5 to 7 day intervals (Mythri 2015).
Blood culture is particularly valuable, as the serological response can be slow or even absent altogether if antibiotics are given early. Isolated leptospires are identified either by serological methods or more recently by molecular techniques The sensitivity of blood cultures is low. Therefore isolation and culture are primarily used for retrospective diagnosis. Urine: Leptospires appear in the urine from about 14th day onwards and the same method of examination as for blood is used. Antibodies may also be present in urine and therefore it is essential to get the sample to the laboratory without delay as the leptospires will die even in alkaline urine. Since urine is acidic and decreases the viability of leptospires, it should be inoculated into the medium within 2 hours after voiding. Viability is reported to be increased in urine samples neutralized with sodium bicarbonate and by using phosphate-buffered bovine serum albumin solution (Mythri 2015).
Cerebrospinal fluid: Leptospires can be isolated by inoculating 0.5ml CSF into 5 ml of the semisolid culture medium during the first weeks of illness.
Indirect evidence:
Serological methods: The wide range of tests that are available are broadly divided into genus specific and serogroup/serotype-specific tests.
Genus specific tests: These tests are based upon the use of a single antigen common for the genus Leptospira. They become positive earlier in the illness and are ideal for a clinical diagnosis. The antigen for these tests is prepared from the non-pathogenic L. biflexa Patoc –1 strain.
Macroscopic slide agglutination test: This test is carried out with a dense suspension of leptospires, which agglutinate into clumps visible to the naked eye. They are performed on slides or plates. Formalin killed antigens are mixed with serum to be tested and viewed with naked eye for presence or absence of agglutination. It allows a provisional diagnosis of acute leptospirosis to be made within a few minutes; It has good correlation with both IgM ELISA and MAT, and therefore can be used as a valuable and simple screening test. The sensitivity of this test can be enhanced by adding the locally-prevalent serovars. It is a simple, rapid, and sensitive diagnostic test for active leptospirosis. It is however not suitable for retrospective testing. Positive reactions, however, should be confirmed by MAT (Mythri 2015).
Indirect fluorescent antibody (IFA) test: Specimens of blood, urine and parenchymatous organs are stained with luminescent sera and examined under a fluorescent microscope. The antigen antibody complex fluoresces brightly and is visible under the microscope. By this method living, dead or even fragmented leptospires can be demonstrated. This test is particularly helpful in the urinary shedders. The test is based on the ability of a specific serum treated with fluorochrome to combine with a specific antigen. This test carries a very high sensitivity and specificity. When the IFA assay was compared with MAT, it was found that the IFA test is moderately sensitive and specific for the initial diagnosis of leptospirosis. It is not dependent on the organisms being intact and lying in the plane of view nor does it require the organisms to be viable as does DFM or culture, but it requires a relatively expensive high quality incident light fluorescence microscope.
Sensitized erythrocyte tests: Leptospiral extracts are used to sensitize sheep and human red blood cells. Cells are mixed with sera containing homologous antibodies. These are sensitized erythrocytes agglutination [SEA] and haemolysing reaction [HL]. The haemolytic test is quite sensitive and adequate in human leptospirosis.6 The same antigen–antibody system is involved in SEA and HL reactions. Compared to the CFT, the HL has the advantage that the complement used in the tests needs to be in excess and therefore it need not be titrated accurately before each test. But it has the disadvantage that the test sera need to be absorbed free from heterophile antibody (Mythri 2015).
Complement fixation test (CFT): CFT is performed using either whole leptospiral cells or soluble extracts. The FT is useful in detecting relatively recent infection. Complement fixation were widely used but methods were not standardised. Complement fixation tests have now been replaced by ELISA methods.
Enzyme linked immuno sorbent assay (ELISA): It is a very sensitive and specific test for the biological diagnosis of leptospirosis. It is of particular value as a serological screening test because of its relative simplicity in comparison with the MAT. ELISA usually detects only the antibodies reacting with a broadly reactive genus-specific antigen and thus gives no indication of the causative serovar or serogroup. They can be performed with commercial kits or with antigen produced “in house”. A broadly reactive so called genus-specific antigen is generally used to detect IgM, and sometimes also IgG antibodies. The presence of IgM antibodies may indicate current or recent leptospirosis, but IgM class antibodies may remain detectable for several years (Mythri 2015).
The detection of specific IgM antibodies could be useful in particular in countries with a high degree of endemicity to help distinguish between acute or recent leptospirosis and past leptospirosis. Stable reagents are available and form the basis of bedside tests, which are read visually. The use of computer assisted automated readers and the appropriate controls improves the reproducibility and predictive value of this test. ELISA methods have been applied in a number of modifications. An IgM specific dot- ELISA was developed in which polyvalent leptospiral antigen was dotted onto nitrocellulose filter disks in microtitre tray wells. A commercial IgM dot ELISA dipstick has been shown to be as sensitive as a microtitre plate IgM ELISA. Assays which detect IgM give positive results earlier in the acute phase of the disease. Immunodot ELISA was comparable with Patoc agglutination for sensitivity, while a similar “line blot” immunoassay was comparable with Patoc agglutination and more sensitive than MAT. A further adaptation of IgM ELISA consists of a dipstick assay using a classical heat-extract of L. biflexa as antigen. Its main appeal is its ease of use, compatible with field conditions encountered where there are few medical resources. This type of test is essentially useful only for screening as it is limited by the same constraints that apply to conventional ELISA methods. A single IgM positive sample taken during an acute febrile illness with symptoms suggestive of leptospirosis can provide presumptive evidence of infection.
9. Treatment
The primary aim of treatment is to control the infection before irreparable damage to the liver and kidneys occurs. Treatment with dihydrostreptomycin as soon as possible after signs appear is recommended.
The results of treatment are often disappointing because in most instances animals are presented for treatment only when the septicemia has subsided. The secondary aim of treatment is to control the leptospiruria of carrier' animals and render them safe to remain in the group. Other Antibiotics used to treat leptospirosis include tetracycline, penicillin, ampicillin, doxycycline, streptomycin and the erythromycin. The efficacy of treatment may depend on the serovar. Fluid therapy, blood transfusion and other supportive care may also be necessary. These supportive treatments depend on the animal and its needs and if the animal is severely affected and in shock it will need fluid therapy.
Fluid therapy is necessary for small animals with fluid losses due to diarrhea and vomiting. Fluids are chosen according to electrolyte imbalances. Blood transfusions are indicated as treatment for the hemolytic anemia in acute leptospirosis in cattle. The clinical indications for a blood transfusion include obvious pallor of the mucous membranes, weakness and tachycardia. In beef herds further abortions may be prevented by vaccination and treatment of all animals with antibiotics and in dairy cattle, only infected animals are usually treated due to the potential loss of milk sale. The primary treatment for equine recurrent uveitis in horses is anti-inflammatory drugs such as corticosteroids and medications to decrease discomfort like topical atropine, Surgery and other therapies may also be used (Yadeta, Bashahun et al. 2016).
TREATMENT THERAPY IS RECOMMENDED BY VEMEDIM
OTC-Fluxin 1ml/10kg body weight, once a day, for 7-10 days consecutive days
Doxylin LA 1ml/10kg body weight, the first 3 doses are 48 hours apart/dose, the last 3 doses are 72 hours apart.
Inject Urotropin 1ml/5-10kg, day/dose, for 7-10 days consecutive days, with antibiotic dose to enhance detoxification and urinary tract antiseptic.
If you see pale pigs, inject more Hemofer 20% + B12, 1-2ml
Supplements: Vimekat 1ml/ 5-10kg body weight, 2-3 days/dose or ATP 1ml/5-10kg body weight
10. Control and prevention of Leptospirosis
10.1 Control and prevention
Interruption of transmission from infected pig or other infected host to susceptible pig is the critical factor in control. Control of leptospirosis is dependent on the combined use of three strategies: antibiotic therapy, vaccination, and management. Unfortunately, not all these options are available in every country. Vaccines are not available in many Western European countries, while problems of antibiotic residues may make the use of anti- biotic therapy difficult in other situations. In the United States, the most useful antibiotic for leptospiral control/ treatment programs, streptomycin, is no longer available for veterinary use. Control programs must therefore be modified to meet local conditions.
Vaccination induces immunity of relatively short duration. Immunity to infection is probably never 100% and, at best, lasts little more than 3 months. Immunity to clinical disease is believed to last somewhat longer, although exact duration is not known. Vaccination markedly reduces the prevalence of infection in swine herds but will not eliminate infection. Improvements in bovine leptospirosis vaccines have resulted in protective immunity lasting approximately 12months. There is a need for the development of similar vaccines for swine that should contain appropriate serovars of pig‐maintained strains plus strains maintained by other regional hosts. Mixed genotype infection of serovar Bratislava indicates that natural infection by one genotype may not always confer immunity to infection by the heterologous genotype of the same serovar (Zimmerman, Karriker et al. 2012).
Antibiotics alone will not eliminate pig‐maintained leptospiral infections from individual carriers or control infection in herds. Despite claims by some authors that either systemic streptomycin at 25mg/kg body weight or oral tetracyclines at levels of 800g/ton of feed will eliminate carriers, others have reported that these regimes do not work. Work on the use of alternative antibiotic therapy regimes indicates that oxytetracycline (40mg/kg for 3 or 5 days), tylosin (44mg/kg for 5 days), or erythromycin (25mg/kg for 5 days) may be effective in eliminating Pomona from the kidneys of experimentally infected pigs.
The main management factor in the control of leptospirosis is the prevention of direct or indirect contact with free‐living vectors or other domestic stock. Strict biosecurity should be implemented, including stringent rodent control programs. When faced with an outbreak of clinical disease, the best option is to treat both affected and at‐risk stock with streptomycin at 25 mg/kg body weight, to immediately vaccinate the at‐risk stock, and then to introduce a regular vaccination program. If vaccination is not an available option, then a feed medication program, using either chlortetracycline or oxytetracycline at 600–800 g/ton of feed, should be introduced. This ration is fed either continuously or on a 1‐month‐on/1‐month‐off basis. Alternatively, it may be fed for two periods of 4 weeks in the year, preferably one in the spring and the other in the autumn. The use of artificial insemination is an important tool in the control of Bratislava infection (Zimmerman, Karriker et al. 2012).
10.2 Vaccination
10.2.1 Recombinant protein vaccines
Recombinant protein vaccines have a great potential against leptospirosis. Several leptospiral recombinant protein vaccines have been constructed with modern biotechnological methods, of which recombinant OMPs, lipoproteins, and virulence factors acquired a considerable interest.
Recombinant OMP vaccines
The protective characteristics of several recombinant OMP vaccines have been tested, including leptospiral outer membrane protein OmpL1, lipoprotein LipL41, hemolysis-associated protein 1 (Hap1) and immunoglobulin-like (Lig) protein. In 1993, the first leptospiral OMP protein, a 31 kDa surface protein OmpL1 of Leptospira, was reported. 6 years later, studies on the Golden Syrian hamster model of leptospirosis demonstrated immunoprotective effects of the leptospiral outer membrane protein OmpL1 and lipoprotein LipL41. However, after another 2 years, it was reported that adenovirus-mediated OmpL1 failed to protect gerbils against heterologous Leptospira infection, contrary to adenovirus-mediated Hap1, which induced a significant protection. In 2002, a 130 kDa immunoreactive leptospiral immunoglobulin-like protein A (LigA) from L. interrogans was described. Then, the immune response of LigA proteins was confirmed, as two immunoglobulin-like proteins, LigA and LigB, induced a protection against leptospires. These results indicated that LigA and LigB may play an important role in the host cell attachment, as well as invasion during leptospiral pathogenesis. Moreover, the lig gene was shown to be useful in the detection of pathogenic Leptospira.
Several leptospiral outer membrane proteins, e.g. LAg42, Loa22, Lk73.5, have been recognized as leptospirosis vaccine candidates, but they were not tested in animal models for vaccine development. LAg42 is a 42 kDa inner-membrane protein. It was identified in pathogenic Leptospira as a factor involved in virulence. Loa22 was found among pathogenic leptospires but not in non-pathogenic leptospires. It is located in the outer membrane and exposed on the cell surface. It has been considered as a candidate for a novel vaccine against leptospirosis. Lk73.5 is a host-inducible immunogenicity protein from pathogenic L. interrogans. Although the protective characteristics of LAg42, Loa22 and Lk73.5 are not available, we believe these outer membrane proteins might be suitable as vaccine candidates (Wang, Jin et al. 2007).
Recombinant lipoprotein vaccines
Lipoproteins are important proteins in leptospires. These proteins are abundant in the outer membrane, to which they are attached through fatty acids. Because of difficulty in production of lipoproteins in heterologous expression systems, only LipL41 was reported as a potential vaccine. However, many lipoproteins (for example: LipL32, LipL45 and LipL21) could be suitable as vaccine candidates.
Recombinant virulence factor vaccines
Only a few papers were reported to identify leptospiral virulence factors, including FlaA, FlaB, Hsp58, SphH and ChpK. FlaA and FlaB are important components of leptospiral periplasmic flagella (PF). PF is a complex structure, composed of a core, surrounded by two sheath layers, which are important virulence factors of Leptospira. In most spirochete species, the core of PF consists of at least three proteins: FlaB1, FlaB2 and FlaB3. The FlaA protein forms a sheath around the FlaB core. FlaA, together with FlaB, impact PF helical morphology.
10.2.2 LPS vaccines
Analysis of LPSs should open new avenues for vaccine developments. The synthesis of LPSs in Leptospira is similar to that in other Gram-negative bacteria. Leptospiral LPSs activate macrophages through CD14 and the Toll-like receptor 2 (TLR2). It is LPS, not lipoprotein, that stimulates the signaling component for macrophages through the TLR2 pathway. Many reports have been published on leptospiral LPSs as vaccine candidates against leptospirosis.
It has been found that a LPS vaccine may be serovar-independent. For example, LPS vaccine prepared from L. biflexa serovar Patoc can effectively protect hamsters against L. interrogans serovar Manilae. In this case, the protective effect strongly depended on the dose and administration times of LPS vaccine prepared from L. biflexa serovar Patoc. However, it was also reported that a LPS vaccine can be serovar-dependent; for example, LPS vaccines prepared from several different serovar strains could not induce a protective immune response in gerbils against the strains of different serovars. Obviously, further studies are required to determine whether other LPS vaccines are serovar-dependent or -independent. If LPS vaccines are serovar-independent in different animals or human, it will make LPS vaccines more simple and efficient (Wang, Jin et al. 2007).
Although LPSs have been tested as leptospirosis vaccines for almost 20 years, and promising results have been obtained, there are still have many problems to be solved. Between others, details of compositions and structures of leptospiral LPSs have to be determined (Wang, Jin et al. 2007).
10.2.3 Inactivated and attenuated vaccines
The inactivated and attenuated vaccines have been reported for more than 50 years. Some inactivated or attenuated leptospirosis vaccines were successfully tested in cattle and dog. Inactivated leptospirosis vaccines were also tested in human volunteers. The sera from persons vaccinated with a bivalent whole cell inactivated vaccine of L. interrogans serovar Hardjo or serovar Pomona contained IgM specific to both serovars. Although the only leptospirosis vaccine licensed for humans is being produced in Cuba since 2006, inactivated and attenuated vaccines still acquire considerable interests. They are especially suitable as veterinary vaccines. When dogs were vaccinated twice with such vaccines and infection with L. interrogans followed the second vaccination, a high rate of protection against L. interrogans was observed and duration of immunity was at least 1-year. The efficiency of inactivated vaccine could be improved by adjuvant and vaccination frequency. A commercial inactivated leptospirosis vaccine (with adjuvant) induced a poor antibody production in cattle during preliminary vaccination. However, after booster vaccination, this vaccine caused a remarkable immune response. Hydrostatic pressure-treated leptospires can be used as inactivated vaccine. After such leptospires were inoculated into rabbits, the vaccine was immunogenic. The inactivated vaccine can induce a strong antigen-specific proliferative response by peripheral blood mononuclear cells (PBMC) of vaccinated cattle 2 months after the first dose of vaccine.
The results discussed above show that inactivated or attenuated vaccines are suitable as the leptospirosis vaccines. However, such vaccines cause safety problems (Wang, Jin et al. 2007).
10.2.4 DNA vaccines
DNA vaccines have been used against different diseases. These vaccines have several advantages over recombinant protein vaccines. Namely, DNA vaccines have very simple processing routes, low prices, and easy administration properties. In just a few years, DNA vaccine technology has been developed from an interesting observation to the practical application. Surprisingly, only two leptospiral DNA vaccine trials have been reported. DNA vaccine encoding hemolysis-associated protein 1 (Hap1) was tested in gerbils, and partial protection against the infection by pathogenic strains of Leptospira was achieved. Another example is the use of a DNA vaccine containing the endoflagellin gene flaB2 in experiments with guinea pigs. Obviously, leptospirosis DNA vaccines need more extensive investigations. It will be very valuable to test the immune characteristics of multiple leptospirosis DNA vaccine complexes in animals (Wang, Jin et al. 2007).
11. References
Bharti, A. R., et al. (2003). "Leptospirosis: a zoonotic disease of global importance." The Lancet infectious diseases 3(12): 757-771.
Cilia, G., et al. (2021). "Insight into the Epidemiology of Leptospirosis: A Review of Leptospira Isolations from “Unconventional” Hosts." Animals 11(1): 191.
Evangelista, K. V. and J. Coburn (2010). "Leptospira as an emerging pathogen: a review of its biology, pathogenesis and host immune responses." Future microbiology 5(9): 1413-1425.
Fearnley, C., et al. (2008). "The development of a real-time PCR to detect pathogenic Leptospira species in kidney tissue." Research in veterinary science 85(1): 8-16.
Frantz, J. C., et al. (1989). "Effect of vaccination with a bacterin containing Leptospira interrogans serovar bratislava on the breeding performance of swine herds." American journal of veterinary research 50(7): 1044-1047.
Guerrant, R. L., et al. (2011). Tropical Infectious Diseases: Principles, Pathogens and Practice E-Book, Elsevier Health Sciences.
Hathaway, S. (1981). "Leptospirosis in New Zealand: an ecological view." New Zealand Veterinary Journal 29(7): 109-112.
Kemenes, F. and T. Süveges (1976). "Leptospira-induced repeated abortion in sows." Acta veterinaria Academiae scientiarum hungaricae 26(4): 395-403.
Ko, A. I., et al. (2009). "Leptospira: the dawn of the molecular genetics era for an emerging zoonotic pathogen." Nature Reviews Microbiology 7(10): 736-747.
Mitchell, D., et al. (1966). "Some observations on the diagnosis and epidemiology of leptospirosis in swine." Canadian Journal of Comparative Medicine and Veterinary Science 30(8): 211.
Mohammed, H., et al. (2011). "Leptospira: morphology, classification and pathogenesis." J Bacteriol Parasitol 2(06).
Mythri, B. (2015). "Laboratory diagnosis of leptospirosis: a review." Journal of Evolution of Medical and Dental Sciences 4(50): 8759-8770.
Pozzi, P. and G. Alborali (2012). "Reproductive diseases in sows (Sus scrofa domestica): a review." Israel Journal of Veterinary Medicine 67(1): 24-33.
Radostits, O. M., et al. (2006). Veterinary Medicine E-Book: A textbook of the diseases of cattle, horses, sheep, pigs and goats, Elsevier Health Sciences.
Saraví, M. A., et al. (1989). "Serological and bacteriological diagnosis, and reproductive consequences of an outbreak of porcine leptospirosis caused by a member of the Pomona serogroup." Revue scientifique et technique (International Office of Epizootics) 8(3): 697-718.
Sellon, D. C. and M. Long (2013). Equine infectious diseases E-book, Elsevier Health Sciences.
Tang, Y.-W. and A. Sails (2014). Molecular medical microbiology, Academic press.
Van Til, L. D. and I. R. Dohoo (1991). "A serological survey of leptospirosis in Prince Edward Island swine herds and its association with infertility." Canadian Journal of Veterinary Research 55(4): 352.
Wang, S., et al. (2020). "Leptospirosis (Weil Disease)." Stat-Pearls. Treasure Island (FL): StatPearls Publishing.
Wang, Z., et al. (2007). "Leptospirosis vaccines." Microbial cell factories 6(1): 1-10.
Yadeta, W., et al. (2016). "Leptospirosis in animal and its public health implications: A review." World Applied Sciences Journal 34(6): 845-853.
Zimmerman, J. J., et al. (2012). Diseases of swine, John Wiley & Sons.
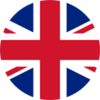