1. Introduction
Feline herpesvirus 1 (FeHV-1) is an alphaherpesvirus that causes feline viral rhinotracheitis, an important viral disease of cats on a worldwide basis. Acute FeHV-1 infection is associated with both upper respiratory and ocular signs. Following the acute phase of the disease lifelong latency is established, primarily in sensory neuronal cells. As is the case with human herpes simplex viruses, latency reactivation can result in recrudescence, which can manifest itself in the form of serious ocular lesions. FeHV-1 infection in cats is a natural host model that is useful for the identification of viral virulence genes that play a role in replication at the mucosal portals of entry or are mediators of the establishment, maintenance, or reactivation of latency. It is also a model system for defining innate and adaptive immunity mechanisms and for immunization strategies that can lead to better protection against this and other alphaherpesvirus infections (Maes, 2012).
2. Aetiology
2.1 Bacterial characteristics
The size of FeHV-1 virions ranges from 120 to 180 nm. They are composed of a core containing the double-stranded viral DNA genome, an icosahedral capsid surrounding the core, a tegument layer surrounding the capsid, and a lipid bilayer envelope from which glycoprotein spikes are protruding (Maes, 2012).
FeHV-1 primarily infects domestic cats, but lions and cheetahs are also susceptible. In vitro, FeHV-1 replicates only in cells of feline origin. Alphaherpesviruses that are genetically related to FeHV-1 are canid herpesvirus 1 (CaHV-1) and phocid herpesviruses (PhHV) 1 and 2 (Maes, 2012).
(A) Reconstruction of a human herpesvirus 1 (HHV-1) capsid generated from cryo-electron microscope images, viewed along the two-fold axis. (B) Schematic representation of a virion with diameters in nm. G: genome; C: capsid; T: tegument; E: envelope. (C) Cryo-electron microscope image of a HHV-1 virion. Structure and assembly of herpesviruses. (D and E) Segmented surface rendering of a single virion tomogram after denoising. (D) Outer surface showing the distribution of glycoprotein spikes (yellow) protruding from the membrane (blue). (E) Cutaway view of the virion interior, showing the capsid (light blue) and the tegument "cap" (orange) inside the envelope (blue and yellow). pp, proximal pole; dp, distal pole
2.2 Classification
Members of the order Herpesvirales comprise a diverse collection of viruses that share characteristic virion morphology. The protein that comes nearest to being herpesvirus-specific is the putative ATPase subunit of the terminase (a complex that is responsible for packaging viral DNA into nascent capsids), which is conserved in all herpesviruses and has more distant relatives in T4-like bacteriophages of the family Myoviridae. The diversity of the order has meant that criteria such as serology or nucleic acid hybridization have limited value in determining relationships between different viruses, and the construction of a satisfactory taxonomic structure has been a significant challenge. Historically, members of the family Herpesviridae were grouped into subfamilies on the basis of broad biological criteria. Division of the subfamilies into genera was based on antigenic cross-reactivity and molecular criteria, primarily the size and structure of the genome. In retrospect, these assignments were found to be generally consistent with sequence-based phylogeny. Modern classification of herpesviruses is based primarily on genetic content (Pellett, 2009).
At all levels from order to species, herpesvirus taxa are described as corresponding to “distinct genetic lineages”; these lineages are defined by two criteria: (a) comparison of nucleotide or predicted amino acid sequences of conserved herpesvirus genes, and (b) identification of particular genes or genetic properties that are unique to a virus subset. Phylogenetic relationships within the family Herpesviridae are illustrated in Figure 2 (Pellett, 2009).
2.3 Genome organization and replication
The number of ORFs contained within herpesvirus genomes that potentially encode proteins ranges from about 70 to more than 200. In addition to proteins, herpesvirus genomes also harbor varying numbers of microRNA genes (none yet identified for some viruses and 33 for HHV-4), and express numerous putatively non-translated transcripts of unknown function. A subset of about 40 protein-coding genes is conserved among the viruses of mammals and birds (family Herpesviridae), arranged into six gene blocks. The conserved gene blocks have different orders and orientations in different herpesvirus subfamilies, but genes within a block generally maintain order and transcriptional polarity. The conserved genes encode capsid proteins, components of the DNA replication and packaging machinery, nucleotide modifying enzymes, membrane proteins and tegument proteins, and to a lesser extent control proteins. This reinforces the view that, despite their genetic diversity, these viruses share common features in many aspects of their replication strategies. Members of the three families in the order Herpesvirales are phylogenetically very distant from each other, detectably sharing only two genes (encoding DNA polymerase and the putative ATPase subunit of terminase) derived from a common ancestor, plus a few additional genes that were probably captured independently. The unifying feature across the order Herpesvirales is virion morphology rather than genetic content.
Given the genetic diversity of members of the order Herpesvirales, it is probable that the details of their replication strategy vary, perhaps substantially. What follows, therefore, is a brief description based on well-studied members of the group, HHV-1 in particular (Figure 4). Adsorption and penetration involve the interaction of multiple virion envelope proteins with multiple cell surface receptors. Entry takes place by membrane fusion either at the cell surface or following endocytosis of the attached virion. The nucleocapsid is transported to the region of a nuclear pore by retrograde microtubule transport, while tegument proteins, many of whose functions are unknown, are thought to modify cellular metabolism. For HHV-1, one tegument protein (the UL41 gene product, vhs) acts in the cytoplasm to inhibit host protein synthesis while another (the UL48 gene product, VP16) is a transcription factor that enters the nucleus and activates viral immediate early genes. In permissive cells, entry of the genome into the nucleus is followed by a transcriptional cascade. Immediate early (α) genes, which are largely distinct among the various families and subfamilies, regulate subsequent gene expression by transcriptional and post-transcriptional mechanisms. Early (β) genes encode the DNA replication complex and a variety of enzymes and proteins involved in modifying host cell metabolism, while the structural proteins of the virus are encoded primarily by late (γ) genes. Immediate early genes can be transcribed in the absence of de novo protein synthesis. Early gene transcription is dependent on expression of immediate early proteins. Late gene transcription is dependent on viral DNA synthesis. With the exception of a small number of non-translated RNAs expressed by specific herpesviruses, transcription is by host RNA polymerase II (Pellett, 2009).
3. Epidemiology
The domestic cat is the main host for FHV, but the virus has also been isolated from cheetahs and lions, and antibodies have been detected in recently in pumas. There is no evidence of human infection.
Latent chronic infection is the typical outcome of an acute FHV infection, and intermittent reactivation gives rise to viral shedding in produced oronasal and conjunctival secretions. Contamination of the environment is not a primary source of transmission, except in catteries. Virus the owner, shedding from acutely infected cats and from latently infected cats experiencing reactivation are the two main sources of infection.
Transplacental infection has not been demonstrated in the field. Latently infected queens may transmit FHV to their offspring because parturition and lactation typically induce stress and may lead to viral reactivation and shedding. Kittens may therefore acquire the virus very early on. The outcome of the infection depends on the level of maternally derived antibodies (MDA) they possess. When high levels are present, kittens are protected against disease and develop subclinical infection leading to latency; in the absence of sufficient MDA, they may develop clinical signs.
In healthy small populations, the prevalence of viral shedding may be lower than 1%, and in large populations, especially when clinical signs are present, it may reach 20%. This low prevalence probably reflects the intermittent nature of viral shedding during latency. In shelters, risks are higher: with 4% of shedders entering the shelter, 50% of cats may excrete the virus 1 week later (Thiry et al., 2009).
3.1 Transmissions
FHV-1 is transmitted to susceptible cats via close contact and transmission of bodily fluids, particularly respiratory and ocular secretions. Cats with a primary infection and upper respiratory-tract disease are most likely to transmit the disease due to the large number of viral particles present in secretions. Infective viral particles can be carried approximately 4 feet by sneezing, thereby exposing nearby cats and dispersing the virus into the environment. Cats with recurrent ocular or respiratory disease may also transmit the virus, although the number of viral particles is probably less in secretions from these cats than in a cat with primary infection and severe upper respiratory-tract disease. Overcrowded conditions and close housing, such as in catteries and shelters, greatly increase the likelihood of viral transmission. Kittens are most susceptible to primary infection, especially as maternal antibodies wane. The virus is short-lived in the environment, remaining viable up to 18 hours in moist conditions and far less in dry conditions. Hands and fomites are vehicles for transmission from handler to cat. The virus is inactivated by most commonly used disinfectants (Stiles, 2003).
4. Pathogenesis
Following primary infection with FHV-1, most cats become latently infected with occasional sub clinical or clinically evident shedding. Recent evidence suggests that extraneural (corneoconjunctival) persistence of virus may also occur in a minority of animals and produce “smoldering” immune-mediated disease.
FHV-1 produces disease by at least two very different mechanisms that require markedly different therapeutic approaches. The first is cytolytic (“cell rupturing”) infection. This can occur during primary infection or following viral reactivation from latency throughout life. Cell rupture is because of active viral replication and therefore therapy may require an antiviral agent. Any form of immunomodulation at this point is probably contraindicated. The clinical manifestations of cytolysis are diverse. Cytolysis can be observed directly in the cornea as ulcerative disease; sometimes in the pathognomonic dendritic formation. However, active viral replication and associated cell rupturing does not always result in loss of all layers of the corneal epithelium and exposure of hydrophilic stroma. Thus, application of fluorescein stain fails to highlight a corneal ulcer at this point and identification of active viral replication may be missed unless rose bengal stain is used to highlight dead and devitalized epithelial cells (Figure 3). Additionally, although viral replication within corneal epithelium and subsequent corneal ulceration receive much clinical attention, FHV-1 preferentially replicates within conjunctiva7 where cell rupturing and sometimes ulceration can be more subtle. However, if cytolysis is severe enough to cause ulceration of mucosal surfaces, serosanguineous ocular discharge can be seen (Figure 5). Simultaneous ulceration of corneal and conjunctival surfaces exposes conjunctival substantia propria and corneal stroma thereby permitting adhesions to form between these tissues (symblepharon; Figure 6). If viral infection occurs before the eyelids open, large amounts of inflammatory debris may accumulate in the conjunctival sac (conjunctivitis neonatorum). Virally mediated cytolysis also causes rhinitis via erosion of nasal mucosa and exposure of underlying bone and cartilage. Subsequent distortion and remodeling of these tissues may be associated with more chronic rhinosinusitis. The second mechanism by which FHV-1 may induce disease is immune-mediated (but not necessarily autoimmune) inflammation. The most striking example of immunopathological herpetic disease in cats is stromal keratitis. This is an uncommon response to infection with FHV-1 seen in only some cats and characterized by infiltration of the corneal stroma with inflammatory cells, especially lymphocytes. Chronic inflammatory changes, especially fibrosis and vascularization, can ultimately cause blindness (Figure 7). Of central importance to the pathogenesis and understanding of stromal keratitis is identification of the antigenic stimulus for this immune response. Strong experimental evidence suggests that persistent viral antigen gains access to the stroma during periods of protracted ulceration and is ineffectually cleared, leading to immune-mediated tissue damage. Regardless of the antigen involved, immune-mediated herpetic disease involves no or low-grade viral replication. This has important clinical implications. Firstly, diagnostic attempts to identify the virus may be unsuccessful and antiviral agents may not be effective as sole therapeutic agents. Secondly, anti-inflammatory therapy may be indicated; usually in addition to antiviral agents (Maggs, 2005).
5. Clinical signs and pathology
5.1 Clinical signs
Table 1: Clinical signs that may assist with differential diagnosis of primary infections with FHV-1 or C. felis in immunologically naïve cats (Maggs, 2005)
Clinical signs | FHV-1 | FCV | C. felis |
Malaise/anorexia | +++ | ++ | ± |
Sneezing | +++ | + | ++ |
Nasal discharge | +++ | ++ | ++ |
Oral ulceration | - | +++ | - |
Ptyalism | + | +++ | ± |
Ocular discharge | +++ | + | ++ |
Conjunctivitis | +++ | - | +++ |
(Hyperemic) | (Chemotic) | ||
Keratitis | +++ | - | - |
The lack of a perfect diagnostic test for FHV-1 has led many to rely on clinical acumen and response to therapy when approaching the etiological diagnosis of feline surface ocular disease. The vast majority of feline keratoconjunctivitis is believed to be infectious in nature; with FHV-1 and Chlamydophila felis (previously Chlamydia psittaci) the major primary pathogens. Calicivirus (FCV) is an unlikely and minor primary conjunctival pathogen and is not a recognized corneal pathogen. Therefore, a few characteristic clinical signs may be useful for differentiating disease resulting from these organisms (Table 1). Acute or primary ocular FHV-1 infection is characterized by conjunctival hyperemia, serous ocular discharge that becomes purulent by day 5 to 7 of infection, mild to moderate conjunctival swelling, and blepharospasm (Figure 5). Primary infection is associated with signs of upper respiratory tract infection. The uncomplicated clinical course is 10 to 21 days. The only pathognomonic clinical sign during primary infection is the presence of dendritic lesions (Figure 4). Milder corneal and/or conjunctival inflammation is typical of recrudescent disease; when seen, dendrites are also considered a pathognomonic clinical feature. Cytologic confirmation of FHV-1 infection based on observation of intranuclear inclusions has not proven useful since the inflammatory cell infiltrate seen in acute FHV-1 infections consists predominantly of neutrophils and is of little diagnostic significance (Maggs, 2005).
This cornea has been stained with rose bengal stain to highlight these dendritic erosions that have not yet caused loss of all epithelial layers and exposure of the underlying corneal stroma (Maggs, 2005).
In this patient the dorsal conjunctiva has adhered to the cornea and symblepharon persists after other signs of active ongoing viral infection have resolved (Maggs, 2005).
Note the corneal stromal edema, inflammatory cell infiltrate and extensive superficial to mid-stromal corneal blood vessels. The epithelium overlying this diseased region of cornea was intact and did not retain fluorescein stain (Maggs, 2005)
5.2 Pathology
Along the airways similar to previously published descriptions, FeHV-1–induced pneumonia was characterized by necrotizing to necrosuppurative bronchitis, bronchiolitis, and alveolitis with occasional intranuclear viral inclusion bodies, neutrophil-dominated leukocyte infiltration, and variable fibrin exudation. The affected cats also exhibited rhinitis and tracheitis of a similar nature (Figure 9-14). Closer examination confirmed that lung lesions were a continuum of those present in the upper respiratory tract. They represented individual focal lesions that typically contained an affected bronchus and bronchiole from which necrosis and inflammation extended into the surrounding alveoli, with frequent evidence of a direct continuation (Figure 11, 12). Immunohistology revealed FeHV-1 antigen in the respiratory epithelial cells of the airways, type I and II pneumocytes, and epithelial cells of the bronchial glands. Viral antigen was primarily seen within the cytoplasm but sometimes also in the nucleus of infected cells (Figure 9b, 11, 12 and 12). Infected epithelial cells showed a range of morphological features, from unaltered cells within mildly affected areas to degenerating cells characterized by cytoplasmic swelling and detached cells with morphological features of apoptosis or necrosis (Figure 10). In addition, viral antigen was occasionally found in alveolar macrophages within alveoli where degenerate infected epithelial cells and free viral antigen were also often present, suggesting that these macrophages had phagocytosed extracellular virions released from dead pneumocytes (Figure 14)
Figure 9. Necrotizing bronchitis, bronchus, case No. 9. (a) The necrotic respiratory epithelium is replaced by a marked neutrophilic inflammatory infiltrate that extends to the bronchial glands, which also exhibit loss of epithelial cells (arrows). Hematoxylin and eosin (HE). (b) FeHV-1 antigen is detected in the necrotic respiratory epithelial cells and cell free in the mucosa and in glandular epithelial cells (arrowhead). Immunohistochemistry (IHC) for FeHV-1 antigen. Figure 10. Necrotizing bronchiolitis, case No. 19. The respiratory epithelium is effaced and replaced by necrotic debris, fibrin, and neutrophils. Asterisk: bronchiolar lumen. HE. Figure 11. Focal necrotizing pneumonia, case No. 4. FeHV-1 infection extends from the bronchus (B) to an associated bronchiole (b) and continues into the alveoli. IHC for FeHV-1 antigen. Figure 12. FeHV-1 spread across the bronchiolo-alveolar transition, case No. 16. Infection stretches from the respiratory epithelial cells (arrow) into the alveoli (A). IHC for FeHV-1 antigen. Figure 13. Necrotizing alveolitis, case No. 18. Alveoli contain necrotic cells and a large amount of fibrin. Inset: Focus with intranuclear inclusion body formation in epithelial cells (arrowheads). HE. Figure 14. Necrotizing alveolitis, case No. 15. The virus infects both type I (long arrows) and type II (short arrow) pneumocytes and possibly also alveolar macrophages (arrowheads). IHC for FeHV-1 antigen.
6. Diagnosis
Virus and antigen detection
The preferred method for virus detection in biological samples is PCR. Virus isolation is still a valid method for detecting infectious FHV but is more time consuming. The sensitivity and specificity of the tests differ between laboratories because there is no standardisation (Thiry et al., 2009).
Detection of nucleic acid
Conventional PCR, nested PCR and real-time PCR are now routinely used by diagnostic laboratories to detect FHV DNA in conjunctival, corneal or oropharyngeal swabs, corneal scrapings, aqueous humour, corneal sequestra, blood or biopsies. Most primers are based on the highly conserved thymidine kinase gene. Molecular methods seem more sensitive than virus isolation or indirect immunofluorescence (Thiry et al., 2009).
Antibody detection
Antibodies to FHV can be detected in serum, aqueous humour and cerebrospinal fluid by serum neutralisation assay or ELISA. Owing to natural infection and vaccination, seroprevalence is high in cats, and the presence of antibodies does not correlate with disease and active infection. Moreover, serology does not distinguish between infected and vaccinated animals. Neutralising antibodies appear 20–30 days after primary infection, and titres may be low, both in cases of acute and chronic disease. Serology, therefore, is of only limited value in the diagnosis of FHV infection (Thiry et al., 2009).
7. Treatment
Supportive treatment
Restoration of fluids, electrolytes and acid–base balance (e.g., replacement of potassium and bicarbonate losses due to salivation and reduced food intake), preferably by intravenous administration, is required in cats with severe clinical signs. Food intake is extremely important. Many cats will not eat because of loss of their sense of smell or ulcers in the oral cavity. Food should be highly palatable and may be blended and warmed up to increase the flavour. Appetite stimulants (e.g., cyproheptadine) may be used. If the cat does not eat for more than 3 days, a feeding tube should be placed. To prevent secondary bacterial infections, broad-spectrum antibiotics that achieve good penetration into the respiratory tract should be given in all acute cases. Nasal discharge should be wiped away using saline and a local ointment. Mucolytic drugs (eg, bromhexine) may be helpful. Eye drops or ointments can be administered several times a day. Nebulisation with saline can be used to combat dehydration of the airways. Vitamins are given, though their value is unclear (Thiry et al., 2009).
Antiviral therapy
Antiviral drugs have been proposed for the treatment of FHV ocular infections, including bromovinyldeoxyuridine, cidofovir, famciclovir, HPMA (N- [2- hydroxy propyl] methacryla mide), penciclovir, ribavirin, valaciclovir, vidarabine, foscarnet and lactoferrin. The efficacy of these drugs is not supported by published data although recent data demonstrate the efficacy of topical ocular application of cidofovir on primary ocular FHV (Thiry et al., 2009).
Vaccination
Feline herpesvirus infection is common and may induce severe, and at times fatal, disease. As a results, FHV was considered to be a core vaccine component and recommends that all cats are vaccinated (Thiry et al., 2009).
8. Control and prevention
Shelters
Feline herpesvirus is a particular problem in cat shelters, and management measures to limit or contain the infection are as important as vaccination. Where incoming cats are mixed with residents, high infection rates ensue. As a rule, therefore, newcomers should be quarantined for 2 weeks and kept individually – unless they are from the same household. Shelter design and management should aim to avoid cross contamination, and new cats should be vaccinated as soon as possible. If there is a particularly high risk (e.g., a recent rhinotracheitis episode), a modified-live virus vaccine is preferable, as it provides earlier protection. If acute respiratory disease is noted, laboratory identification of the agent with differentiation between FHV and FCV can be useful in designing appropriate preventive measures (Thiry et al., 2009).
Breeding catteries
In breeding catteries, FHV can cause major problems. The infection surfaces most often in young kittens before weaning, typically at around 4–8 weeks of age, as MDA wane. The virus source is often the mother whose latent infection (carrier state) has been reactivated following the stress of kittening and lactation. Clinical signs can be severe and frequently involve all kittens in the litter. Mortality can occur, and some recovered kittens are left with chronic rhinitis. Vaccination of the queen will not prevent this problem, because it will not prevent her from becoming a carrier. However, if she has a high antibody titre, the kittens will benefit from MDA in the colostrum, which should provide protection for the first weeks of life. Booster vaccinations of queens may therefore be indicated and should be given before mating. Vaccination during pregnancy may be considered only as an exception. Feline herpesvirus vaccines are not licensed for use in pregnant cats and an inactivated product may be preferable in these cases.
Breeding management plays a crucial role in controlling FHV in catteries. Queens should kitten in isolation, and their litters should not mix with those of other cats until they have been fully vaccinated. Early vaccination should be considered for litters from queens that have had infected litters previously. The earliest age for which FHV vaccines are licensed is 6 weeks, but vaccination from around 4 weeks of age may be considered (kittens are already immunocompetent at that age), with repeated injections every 2 weeks until the normal primary vaccination course is started (Thiry et al., 2009).
9. References
King, A.M., Lefkowitz, E., Adams, M.J., Carstens, E.B., 2011. Virus taxonomy: ninth report of the International Committee on Taxonomy of Viruses. Elsevier.
Maes, R., 2012. Felid herpesvirus type 1 infection in cats: a natural host model for alphaherpesvirus pathogenesis. ISRN veterinary science 2012, 495830.
Maggs, D.J., 2005. Update on pathogenesis, diagnosis, and treatment of feline herpesvirus type 1. Clinical techniques in small animal practice 20, 94-101.
Pellett, P.E.C., Davison, A.J., Eberle, R., Ehlers, B., Hayward, G.S., Lacoste, V., Minson, A.C., Nicholas, J., Roizman, B., Studdert, M.J. and Wang, F., 2009. Herpesvirales, ICTV Ninth Report; 2009 Taxonomy Release. ICTV.
Stiles, J., 2003. Feline herpesvirus. Clinical techniques in small animal practice 18, 178-185.
Thiry, E., Addie, D., Belák, S., Boucraut-Baralon, C., Egberink, H., Frymus, T., Gruffydd-Jones, T., Hartmann, K., Hosie, M.J., Lloret, A., 2009. Feline herpesvirus infection. ABCD guidelines on prevention and management. Journal of Feline Medicine & Surgery 11, 547-555.
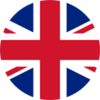