Truong Minh Hieu, Nguyen Khanh Thuan, Nguyen Phuc KHanh, Nguyen Thanh Lam*
Tran Duy Thanh, Pham Trang Thanh Nguyen
1. Introduction
Fowl cholera (FC) (avian cholera, avian pasteurellosis, or avian hemorrhagic septicemia) is a contagious disease affecting domesticated and wild birds. It usually appears as a septicemic disease associated with high morbidity and mortality, but chronic or benign conditions often occur. The majority of acute fowl cholera cases are caused by serogroup A strains of Pasteurella multocida. In the acute form of the disease, clinical signs may only be present shortly before death. Mortalities range up to 20%, although even higher rates are reported. In the chronic form of the disease, clinical signs are typically related to localized infections with wattles, sinuses, leg or wing joints, foot pads, and sternal bursae often enlarged. Both commercial and autogenous bacterins are widely used to reduce the economic losses associated with fowl cholera. In some areas, live vaccines are available. Sensitivity testing should be done to guide any antimicrobial use (David E. Swayne, 2019).
2. Aetiology
2.1 Classification
The genus Pasteurella was named in honor of the famous French microbiologist and chemist Louis Pasteur, who first isolated this bacterium in the 1880s as the causative agent of “choléra des poules” (fowl cholera). Since that time, Pasteurella species have been linked as causative agents of many infectious diseases in a wide range of animals as well as in humans. Pasteurella multocida is the main causative agent of FC. Like most Pasteurella species, P. multocida is often found as part of the normal oropharyngeal microbiota of many animals and birds but can also be a primary or opportunistic pathogen of the upper and lower respiratory tracts. Three subspecies of P. multocida were formally recognized in 1985, P. multocida subsp. multocida, subsp. gallicida, and subsp. septica (Peng et al., 2019).
Traditionally, P. multocida isolates have been serologically classified into five capsular serogroups (A, B, D, E, and F) and 16 lipopolysaccharide (LPS) serovars (serovar 1 through serovar 16), using methods developed by Carter in 1952 and Heddleston et al. in 1972, respectively. These serological typing methods have been widely used in epidemiological studies to determine the serovars of P. multocida strains that are circulating in different host species and that are associated with different types of diseases. However, these two serological typing methods require high-quality antisera, which are difficult to prepare and implement for clinical use. Therefore, these serological methods are not commonly used any longer for typing P. multocida isolates in many laboratories. Instead, more rapid, accurate, and reproducible molecular sequence typing methods have become more popular for diagnostics. The most frequently used molecular typing methods for P. multocida are capsular genotyping, LPS genotyping, MLST, and/or virulence genotyping based on the detection of different virulence gene profiles. The capsular genotyping method was developed based on PCR detection of cap genes, using primers highly specific for different serogroups: hyaD-hyaC for serogroup A, bcbD for serogroup B, dcbF for serogroup D, ecbJ for serogroup E, and fcbD for serogroup F. The genotypes determined by this multiplex capsular PCR-based typing system reliably and accurately discriminate among the capsular serogroups.
2.2 Morphology
P. multocida is a Gram-negative, nonmotile, nonspore-forming rod occurring singly, in pairs, and occasionally as chains or filaments. It measures 0.2–0.4 × 0.6–2.5mm but tends to become pleomorphic after repeated subculture. A capsule can be demonstrated in recently isolated cultures using indirect methods of staining (Figure 2). In tissues, blood, and recently isolated cultures, the organism stains bipolar (Figure 3). Pili have been reported (Glorioso et al., 1982; Rebers et al., 1988).
P. multocida strains can be cultured on rich medium, such as tryptic soy agar, chocolate agar, or brain heart infusion agar, supplemented with 5 to 10% fetal calf serum or horse or sheep blood, under aerobic conditions at 37°C; they are oxidase and catalase positive; and they can ferment many carbohydrates, such as fructose, glucose, mannose, and galactose, to produce acid but not gas. Biochemical tests can be used for preliminary differentiation of P. multocida subspecies, where P. multocida subsp. multocida isolates are generally dulcitol negative and sorbitol positive, P. multocida subsp. Gallicida isolates are generally dulcitol positive and sorbitol negative, and P. multocida subsp. septica isolates are generally dulcitol negative and sorbitol negative.
2.3 Bacterial resistance
Pasteurella multocida is destroyed easily by ordinary disinfectants, sunlight, drying, or heat; it is killed within 15 minutes at 56°C and 10 minutes at 60°C. A 1% solution of formaldehyde, phenol, sodium hydroxide, betapropiolactone, or glutaraldehyde and a 0.1% solution of benzalkonium chloride all killed, within 5 minutes, a suspension of 4.4 × 108 organisms of P. multocida/mL in 0.85% saline solution at 24°C.
Skidmore (1932) observed that the organism survived in dried turkey blood on glass for 8 days but not 30 days at room temperature. In studies of environmental influence on the incidence of FC, Van Es and Olney (1940) found the infection hazard had apparently disappeared from a poultry yard 2 weeks after the last death and removal of birds.
The influence of temperature on the viability and virulence of P. multocida was studied by Nobrega and Bueno (1950), who observed that broth cultures stored in sealed tubes at an average room temperature of 17.6°C were still virulent after 2 years; at 2°C–4°C, while they were nonviable after 1 year. With controlled experiments, Dimov (1964) observed that P. multocida died rapidly in soils with moisture content of less than 40%. At a moisture content of 50% and temperature of 20°C, it survived for 5–6 days at pH 5.0, 15–100 days at pH 7.0, and 24–85 days at pH 8.0. A culture survived without loss of virulence for 113 days in soil with 50% moisture at 3°C and pH 7.15. Cultures may be maintained without dissociation or loss of virulence in the lyophilized state or sealed in glass tubes and stored at 4°C or colder for up to 12 months (Watko, L.P. and Heddleston K.L., 1966).
3. History of disease
Several epornitics among fowl occurred in Europe during the latter half of the 18th century. The disease was studied in France in 1782 by Chabert and in 1836 by Mailet, who first used the term fowl cholera. Huppe in 1886 referred to hemorrhagic septicemia, and Lignieres in 1900 used the term avian pasteurellosis. Benjamin in 1851 gave a good description of the disease and demonstrated that it could be spread by cohabitation. With this knowledge of the disease, he formulated procedures for its prevention. At about the same time, Renault, Reynal, and Delafond demonstrated its transmissibility to various species by inoculation. In 1877 and 1878, Perroncito of Italy and Semmer of Russia observed in tissues of affected birds a bacterium that had a rounded form and occurred singly or in pairs. In 1879, Toussaint isolated the bacterium and proved it was the sole cause of the disease.
Pasteur (1880) isolated the organism and grew pure cultures in chicken broth. In further studies, Pasteur (1881) used the FC organism to perform his classic experiments in attenuation of bacteria for use in producing immunity. Salmon (1880) appears to have been the first to study the disease in the United States. A good description of disease signs was reported, however, as early as 1867 in Iowa, where losses of chickens, turkeys, and geese had occurred (David E. Swayne, 2019).
4. Epidemiology
4.1 Incidence and distribution
Normal fowl cholera is more prevalent in late summer, fall, and winter. This seasonal occurrence is one of circumstance rather than lowered resistance, except that chickens become more susceptible as they reach maturity (David E. Swayne, 2019).
4.2 Natural and experimental hosts
Most reported outbreaks of FC affected chickens, turkeys, ducks, or geese. However, this disease also affects other types of poultry, game birds raised in captivity, companion birds, birds in zoos, and wild birds. The wide range of avian hosts in which FC has been reported suggests that all types of birds are susceptible.
Among types of poultry, turkeys are most affected. Most or all in an infected flock may die within a few days. The disease usually occurs in young mature turkeys, but all ages are highly susceptible. Under experimental conditions, 90%–100% of mature turkeys may die within 48 hours when exposed to a highly virulent strain of P. multocida by swabbing the palatine cleft or by contact with infected birds.
The disease in turkeys was first reported in detail by DeVolt and Davis (1932), who described an outbreak in a flock of 175 turkeys in Maryland, where the mortality was 17%. Alberts and Graham (1948) described outbreaks in 4 flocks of turkeys in which mortality was 17%–68%. They emphasized that environmental stressors such as changes in climate, nutrition, injury, and excitement may have influenced the incidence and course of the disease.
Death losses from FC in chickens usually occur in laying flocks, because birds of this age are more susceptible than younger chickens. Chickens less than 16 weeks of age generally are quite resistant. However, the disease has been seen in broiler chickens. Fowl cholera in young chickens usually is caused by serotype 1 and often occurs in conjunction with some other malady. Experimental challenge of 5-week-old broilers with 2 representative strains (serotypes 3 and 1,3) resulted in mortality and lameness.
In naturally infected chickens, mortality usually ranges from 0%–20%, but greater losses have been reported. Reduced egg production and persistent localized infection often occur. Chickens are more susceptible to FC after withdrawal of feed and water or after an abrupt change of diet. Heat or rough treatment on a shaking machine increased the incidence in chickens exposed experimentally. Under experimental conditions, 90%–100% of mature chickens exposed by swabbing the palatine cleft may die within 24–48 hours, depending on the strain of P. multocida used, but only 10%–20% usually die within a 2-week period when exposed by contact with infected birds. Pritchett et al. (1930) observed mortality of 35%–45% in 3 houses of pullets. In majority of areas, FC existed mainly as a persistent, subacute chronic disease that clinically resembles avian monocytosis (David E. Swayne, 2019).
Domestic geese and ducks are also highly susceptible to FC. Van Es and Olney recognized the marked susceptibility of geese to FC, in using them to test for persistence of viable organisms in lots after removal of infected chickens. FC in ducks has been a serious problem on Long Island, where it was diagnosed on 32 of 68 commercial duck farms. Birds of prey, waterfowl, and other birds kept in zoologic gardens occasionally succumb to infection; P. multocida has been isolated from more than 50 species of feral birds. During a 2.5‐year survey, Faddoul et al. isolated P. multocida from 13 (7 species) of 248 feral birds submitted to the diagnostic laboratory.
4.3 Transmission, carriers and vectors
How FC is introduced into a flock is often impossible to determine. Chronically infected birds are considered to be a major source of infection. The only limit to the duration of the chronic carrier state is the life span of the infected bird. Free-flying birds having contact with poultry may be a source of FC organisms. Transmission of the organism through the egg seldom, if ever, occurs. A study of more than 2,000 fresh and embryonating eggs from chickens infected with chronic FC yielded no evidence that P. multocida was transmitted through the egg (David E. Swayne, 2019).
Acute pasteurellosis infections are common in worldwide and they can cause bird deaths in 12 h, albeit 24–48 h is typical. Vulnerability to contamination and the formation of malady depends on various factors, including gender, age, and hereditary variety. Many birds harbor the organism in nasal clefts. The presence of the bacterium is generally related to severity of upper respiratory infection in the birds. The enzootic focus of infection is healthy nasal carriers. Transmission to vulnerable birds from contaminated wetlands or from direct bird-to-bird contact is the in all probability routes of transmission amid epizootics (Figure 4). Two field cultures were isolated from raccoons that were pathogenic for poultry. Sparrows and pigeons carried organisms without showing clinical signs, but 10% of infected rats developed acute pasteurellosis. The possibility that insects may serve as vectors of FC has been investigated. Transmission by flies, however, is probably not common, as indicated by previous studies. Although FC was maintained in two lots of chickens during the height of the fly season, no spread of the disease occurred to adjoining lots separated only by bird nesting. It was observed that larvae, nymphs, and adult ticks (Argas persicus) contained P. multocida after feeding on infected hens. A previous demonstration described that the red mite (Dermanyssus gallinae) became infected with P. multocida after feeding on infected birds, but the mite did not transmit the organism (Parın et al., 2018).
Dorsey and Harshfield (1959) reported a higher incidence of FC during late summer and fall in South Dakota. Carrier birds among the older flock, held over for a second year, provided a reservoir of infection for young susceptible pullets housed with them.
Most species of farm animals may be carriers of P. multocida. Generally, these organisms, except for those from swine and possibly those from cats, are avirulent for fowl. Iliev et al. (1963) isolated P. multocida from tonsils of 34 of 75 slaughtered cattle, 14 of 27 sheep, and 102 of 162 pigs. Isolates from cattle and sheep were not pathogenic for fowl, but all 18 isolates from pigs in areas where FC was common were highly pathogenic for fowl. Only 2 of 47 isolates from pigs in areas having low incidence of FC were pathogenic. Iliev et al. (1965) also reported that healthy pigs that were carriers of P. multocida transmitted infection to fowl in the same enclo-sure. Two isolates, serotypes 1:A and 5:A, from lungs of pigs with pneumonia, were studied by Murata et al. (1964). Serotype 5:A was highly virulent for chickens, and serotype 1:A was avirulent. They found no cross-immunity in chickens between the 2 serotypes.
Gregg et al. (1974) isolated 2 cultures from raccoons that were pathogenic for turkeys. They suggested that raccoons are a reservoir of P. multocida, and the organisms may be transmit- ted to turkeys via the raccoon bite. Contaminated crates, feed bags, or any equipment used previously for poultry may serve in introducing FC into a flock. Organisms are disseminated throughout the carcasses of birds that die of acute FC and may serve as an infection source, especially because fowl tend to consume such carcasses. Hendrickson and Hilbert (1932) were able to isolate P. multocida from the blood of a naturally infected chicken for 49 days preceding death. They noticed a rapid increase in the number of organisms immediately preceding and following death and that the organisms remained viable for 2 months at 5oC–10oC. Serdyuk and Tsimokh (1970) demonstrated experimentally that sparrows, pigeons, and rats could become infected with P. multocida when exposed to chickens with FC and that they in turn could infect susceptible chickens. Sparrows and pigeons carried organisms without showing clinical signs, but 10% of infected rats developed acute pasteurellosis.
The possibility that insects may serve as vectors of FC has been investigated. Skidmore (1932) experimentally transmitted FC to turkeys by feeding them flies that had previously fed on infected blood. He pointed out that under natural conditions, ingestion of flies might be a means of introducing the disease into a flock. Transmission by flies, however, is probably not common, as indicated by studies of Van Es and Olney (1940). Although FC was maintained in 2 lots of chickens during the height of the fly season, no spread of the disease occurred to adjoining lots separated only by poultry netting. Iovcev (1967) observed that larvae, nymphs, and adult ticks (Argas persicus) contained P. multocida after feeding on infected hens. Petrov (1975) demonstrated that the red mite (Dermanyssus gallinae) became infected with P. multocida after feeding on infected birds, but the mite did not transmit the organism.
Heddleston and Wessman (1963) showed that 27 cultures of P. multocida from the upper respiratory tract of humans were not pathogenic for turkeys. Humans can become infected, however, and may infect poultry via excretion from the nose or mouth.
Dissemination of P. multocida within a flock is primarily by excretions from the mouth, nose, and conjunctiva (Figure 5) of diseased birds that contaminate their environment, particularly feed and water. Feces very seldom contain viable P. multocida. Turkeys drinking from the same water through with those experimentally infected with P. multocida developed FC (Pabs‐Garnon, L. and M.A. Soltys, 1971).
5. Pathogenesis
The pathogenicity of P. multocida is complex and variable, depending on the strain, host species, and variations within the strain or host and conditions of contact between the strain and the host.
P. multocida usually enters tissues of birds through mucous membranes of the pharnyx or upper air passages, but it also may enter through the conjunctiva or cutaneous wounds. Hughes and Pritchett (1930) were unable to infect chickens by placing a culture in a gelatin capsule and inserting it into the esophagus, but chickens were infected when the culture was dropped on the roof of the nasal cleft. Arsov (1965) infected birds by mouth, using 35P-labeled culture, and observed that the portal of infection was the mucous membrane of the mouth and pharnyx but not the esophagus, crop, or proventriculus. The Eustachian tube was suggested by Olson and McCune (1968) as the most likely route of infection because the infection localizes in air spaces of the cranial bone, middle ear, and meninges.
Following colonization of the upper respiratory tract by pathogenic P. multocida strains invasion and septicaemia usually develop. Matsumoto et al. (1991) showed that spread to the lungs with subsequent multiplication may occur before the organism invades the bloodstream. P. multocida may also enter the tissues through the conjunctiva or cutaneous lesions and result in localized cutaneous wounds or septicaemia. The fully virulent organism has been reported to multiply rapidly either in the bloodstream or initially in the liver and spleen before being released into the bloodstream at the terminal phase of infection. The cause of death is assumed to be endotoxic shock and signs of acute fowl cholera have been reproduced by injection of endotoxin from P. multocida (J. P. Christensen and M. Bisgaard 1997).
The ability of P. multocida to invade and reproduce in the host is enhanced by the presence of a capsule that surrounds the organism. Loss of ability of a virulent strain to produce the capsule results in loss of virulence. Many isolates from cases of fowl cholera have large capsules but are of low virulence. Therefore, virulence is apparently related to some chemical substance associated with the capsule, rather than with its physical presence.
Turkeys are much more susceptible than chickens to infection with P. multocida, and mature chickens are more susceptible than young ones. Hungerford (1968) observed heavy losses in mature chickens, but no losses in birds up to 16 weeks of age in a case involving 90,000 birds. When testing infectivity of an isolate or susceptibility of a host, cohabitation is the most natural method of exposure. Unless the host is highly susceptible and the isolate highly invasive, however, results may be slow. Therefore, it is often advantageous to swab the nasal cleft with cotton saturated with the culture; if a more severe exposure is required, the culture can be injected parenterally. (David E. Swayne, 2019).
6. Virulence factors
Although no definitive and complete understanding of the virulence of P. multocida is currently available. The capsule of P. multocida has long been regarded as an important virulence factor. Recent work has confirmed the key role of the capsule with a genetically defined, acapsular mutant of a serogroup A strain being shown to be markedly attenuated in both mice and chickens, and also unable to grow in chicken muscle.
There is clear evidence of the role of LPS in the virulence of P. multocida. The LPS of P. multocida consists of a highly conserved inner core that is linked to lipid A and an outer core that shows considerable variation with no polymeric O side chain. A series of studies have confirmed that the 16 Heddleston serovars do represent unique LPS structures. Studies using either the Heddleston serovar reference strains or VP161, a strain from a FC outbreak in Vietnam, have confirmed that apparently minor changes in the LPS structure can have major impacts on virulence: for example, decoration of the LPS with phosphocoline is important although not essential for virulence; truncating the LPS by inactivating a heptosyltransferase removes virulence; and that mutants expressing only inner glycoform B are avirulent whereas the parent with both forms (the wild type) or a mutant expressing only glycoform A were fully virulent.
The other potential virulence‐linked genes are typically those associated with fimbrae/adhesins (e.g, fimA, flp1, flp2, hsf_1, hsf_2, pfhA, and ptfA), iron regulated and iron acquisition proteins (e.g., tbpA, tonB and exbBD), sialic acid metabolism (nanB, nanH), outer membrane proteins (e.g., oma87, ompH) and global regular genes (e.g., crp and phoP) (David E. Swayne, 2019).
7. Toxicity
A dried culture filtrate of P. multocida was first demonstrated to produce signs of toxicity in chickens by Pasteur (1880a). Salmon (1880) repeated this work and described signs resulting from toxicity similar to those observed in cases of acute FC. Kyaw (1944), using the developing chick embryo in the study of pathogenesis, suggested that a toxin was produced in vivo by P. multocida. Rhoades (1964) observed severe general passive hyperemia in chickens that died from acute FC. This lesion was considered to be indicative of shock and was attributed to the action of endotoxin.
7.1 Endotoxins
Endotoxins are produced by all P. multocida, both virulent and nonvirulent. They may contribute to virulence; however invasion and multiplication of a strain are necessary for the production of sufficient quantities of endotoxin in vivo to contribute to pathologic processes.
Pirosky (1938) obtained an endotoxin from P. multocida of avian origin by the trichloroacetic acid extraction procedure of Boivin et al. Heddleston and Rebers (1975) demonstrated that a loosely bound endotoxin could be washed from P. multocida with cold formalinized saline solution. This endotoxin was a nitrogen-containing phosphorylated lipopolysaccharide, readily inactivated under mild acid conditions. Signs of acute FC were induced in chickens by injection of fractional amounts of endotoxin. The LD50 for chicken embryos was 5.2mg via the chorioallantoic membrane; the LD50 for mice was 194 mg via the peritoneal cavity. One dose of 1.9mg injected intravenously killed 5 of 6 19-day-old turkeys; the median death time was only 3 hours. The endotoxin was present in the vascular system of turkeys with FC and could be detected with the Limulus lysate test and antiserum in the gel diffusion precipitin test. The serologic specificity of the endotoxin was associated with the lipopolysaccharide. Free endotoxin induced active immunity.
Purified lipopolysaccharides of each of the Heddleston serotypes were prepared by Rimler et al. (1984). The lipopolysaccharides were similar to those of other Gram-negative bacteria. Week-old poults were relatively resistant to the lethal effects of purified lipopolysaccharides from 2 highly pathogenic FC strains of P. multocida (1987). In poults, the lipopolysaccharides did not provoke a dermal Shwartzman reaction, and lethality was not enhanced by a liver-damaging substance, a histamine-releasing substance, or a surgical bursectomy.
7.2 Protein toxins
Heat-labile protein toxins have been found in serogroup A and D strains isolated from different animal species. Nielsen et al. (1986) found 6 of 10 turkey strains produced heat-labile protein toxins; the strains were not serotyped. Four serogroup D strains isolated from turkeys were found to contain a heat labile toxin. Sonicated suspensions of these strains produced necrotic lesions in turkey skin and were lethal to poults. Antiserum prepared against the heat-labile toxin from a swine strain neutralized the ability of the avian strain sonicated material to produce skin necrosis. Baba and Bito (1966) chemically purified a protein toxin from an avian strain.
8. Clinical signs of disease and pathology
8.1 Clinical signs
Acute disease
Signs of infection in acute FC are often present for only a few hours before death. The acute form is very rapid and signs may only be present for a few hours before death including fever, anorexia, ruffled feathers, mucous discharge from the beak, diarrhea, increased respiratory rate, and cyanosis just prior to death. Geese may just die acutely with no premonitory signs. Unless infected birds are observed during this period, death may be the first indication of disease. Signs that often occur are fever, anorexia, ruffled feathers, mucous discharge from the mouth, diarrhea, and increased respiratory rate. Cyanosis often occurs immediately prior to death and is most evident in unfeathered areas of the head, such as comb and wattles. Fecal material associated with the diarrhea is initially watery and whitish in color but later becomes greenish and contains mucus. Birds that survive the initial acute septicemic stage may later succumb to the debilitating effects of emaciation and dehydration, may become chronically infected, or may recover.
Chronic disease
The chronic form can occur following an acute stage or be from low-virulence organisms. It consists of localized infections whose site(s) determine the type of clinical signs, which include swollen wattles, sinuses, joints, foot pads, and sternal bursae; exudative conjunctivitis and pharyngitis; torticollis; tracheal rales; dyspnea; and roup and bloody discharge from the beak. Chronic FC may follow an acute stage of the disease or result from infection with organisms of low virulence. Signs generally are related to localized infections. Wattles (Figure 6), sinuses, leg or wing joints, foot pads, and sternal bursae often become swollen. Exudative conjunctival (Figure 7) and pharyngeal lesions may be observed, and torticollis (Figure 8) sometimes occurs. Tracheal rales and dyspnea may result from respiratory tract infections. In the past, the term roup was used to indicate a condition in which signs were associated with chronic infections of cephalic mucous membranes. The term was not limited to FC, but included other diseases as well. Chronically infected birds may succumb, remain infected for long periods, or recover.
Morbidity and mortality
In naturally infected chickens, mortality usually ranges from 0% to 20%, but greater losses have been reported. Reduced egg production and persistent localized infection often occur. Chickens are more susceptible to FC after feed and water withdrawal or after an abrupt change of diet. Heat or rough treatment on a shaking machine increased the incidence in chickens exposed experimentally. The move to organic and free‐range production systems has resulted in some changes in disease expression. In a free‐range organic broiler flock, an ongoing FC outbreak caused mortalities of 55% through to processing. Under experimental conditions, 90%–100% of mature chickens exposed by swabbing the palatine cleft may die within 24–48 hours, depending on the strain of P. multocida used. In contrast, only 10%–20% usually die within a 2‐week period when exposed by contact with infected birds. Pritchett et al. (1930) observed mortality of 35%–45% in 3 houses of pullets. In South Carolina and adjoining areas, FC existed mainly as a persistent, subacute chronic disease that clinically resembles avian monocytosis. Most or all turkeys in an infected flock may die within a few days. Under experimental conditions, 90%–100% of mature turkeys may die within 48 hours when exposed to a highly virulent strain of P. multocida by swabbing the palatine cleft or by contact with infected birds. An FC outbreak in geese in Rhode Island resulted in about 3,200 of a flock of 4,000 dying in a short period. Losses usually occur in ducks greater than 4 weeks of age, with mortality reaching 50%. Fowl cholera outbreaks in wild birds are often associated with large mortalities. Jaksic et al. (1964) described an acute epornitic among pheasants, in which 1,700 died. An FC outbreak in the San Francisco Bay area was reported to have been responsible for an estimated loss of 40,000 waterfowl. Gershman et al. (1964) observed a serious outbreak among eider ducks (Somateria mollissima) in a nesting area 6 miles off the coast of Maine, where more than 200 birds died and more than 100 nests were lost. More than 60,000 waterfowl died of FC during the winter of 1956–1957 at the Muleshoe National Wildlife Refuge in Texas. Rosen (1971) reported that there are 2 areas in the United States where FC is enzootic in waterfowl: the Muleshoe National Wildlife Refuge and the north central area of California. Both locations have had periodic outbreaks since 1944 (David E. Swayne, 2019).
8.2 Pathology
Lesions of FC are not constant but vary in type and severity. The greatest variation is related to the course of the disease, whether acute or chronic. Although it is convenient for descriptive purposes to refer to either acute or chronic FC, it is sometimes difficult to categorize the disease in this manner. Signs of infection and lesions that occur may be intermediate to those described for acute and chronic forms.
Gross pathology of acute disease
When the course of the disease is acute, most of the postmortem lesions are associated with vascular disturbances. General hyperemia usually occurs, is most evident in veins of the abdominal viscera, and may be quite pronounced in small vessels of the duodenal mucosa (Figure 9). Large numbers of bacteria usually can be observed microscopically in the hyperemic vessels. Petechial and ecchymotic hemorrhages are frequently found and may be widely distributed. Subepicardial (Figure 10A) and subserosal hemorrhages are common, as are hemorrhages in the lung, abdominal fat, and intestinal mucosa. Increased amounts of pericardial and peritoneal fluid frequently occur. Disseminated intravascular clotting or fibrinous thrombosis has been observed in chickens and ducks that died from acute experimentally induced FC.
Livers of acutely affected birds may be swollen and usually contain multiple small focal areas of coagulative necrosis (Figure 10B) and heterophilic infiltration. Some of the less virulent P. multocida do not produce necrotic foci in the liver. Heterophilic infiltration also occurs in lungs and certain other parenchymatous organs. Lungs of turkeys are affected more severely than those of chickens, with pneumonia being a common sequela. Large amounts of viscid mucus may be observed in the digestive tract, particularly in the pharynx, crop, and intestine.
Ovaries of laying hens are commonly affected. Mature follicles often appear flaccid; thecal blood vessels, which are usually easily observed, are less evident (Figure 10E). In some hens, the blood vessels of the ovaries will be very prominent. Yolk material from ruptured follicles may be found in the peritoneal cavity. Immature follicles and ovarian stroma are often hyperemic (David E. Swayne, 2019).
Gross pathology of chronic disease
Chronic FC is characterized by localized infections, in contrast to the septicemic nature of the acute disease. These generally become suppurative and may be widely distributed anatomically. They often occur in the respiratory tract and may involve any part, including sinuses and pneumatic bones. Pneumonia (Figure 10C, D) is an especially common lesion in turkeys. Infections of the conjunctiva and adjacent tissues occur, and facial edema may be observed. Localized infections also may involve the hock joints (Figure 10F), foot pads, peritoneal cavity, and oviduct.
Chronic localized infections can involve the middle ear and cranial bones and have been reported to result in torticollis. In turkeys, torticollis and eventual death can be associated with infections of the cranial bones, middle ear, and meninges. In a study of naturally infected turkeys exhibiting torticollis, Olson (1966) described lesions at these sites. The outstanding gross lesion was yellowish caseous exudate in air spaces of the calvarial bones. Heterophilic infiltration and fibrin were consistently observed in the air spaces, middle ear, and meninges. Multinuclear giant cells often were associated with necrotic masses of heterophils in air spaces. Similar lesions were found in experimentally exposed turkeys. Localized meningeal infections, without involvement of cranial bones or the middle ear, have been observed in turkeys exhibiting torticollis, as have cerebellar infections. (David E. Swayne, 2019).
Microscopic pathology of acute disease
Experimental infection of mature chickens resulted in an acute general passive hyperemia with heterophilic infiltration of the lung, liver, and other organs (Figure 11). In experimental infections of ducks, the acute disease presents as a hemorrhagic septicemia with widespread vascular damage and focal necrosis in liver, spleen, and other organs (David E. Swayne, 2019).
Microscopic pathology of chronic disease
In a study of naturally infected turkeys exhibiting torticollis, Olson (1966) described lesions in cranial bones, middle ear, and meninges. The outstanding gross lesion was yellowish caseous exudate in air spaces of the calvarial bones. Heterophilic infiltration and fibrin were consistently observed in the air spaces, middle ear, and meninges. Multinuclear giant cells often were associated with necrotic masses of heterophils in air spaces. Similar lesions were found in experimentally exposed turkeys. Localized meningeal infections (Figure 12), without involvement of cranial bones or the middle ear, have been observed in turkeys exhibiting torticollis, as have cerebellar infections (David E. Swayne, 2019).
9. Diagnosis
A presumptive diagnosis of FC may be made from clinical observations, necropsy findings, or isolation of P. multocida. A conclusive diagnosis should be based on all 3. Signs and lesions of the disease were described previously.
9.1 Isolation and identification
P. multocida can be isolated readily from viscera of birds that die of acute FC and usually from lesions of chronic cases; it is less likely to be isolated from dehydrated, emaciated survivors of an acute outbreak. A tentative diagnosis of acute FC can be made by demonstrating bipolar organisms in liver imprints using Wright’s stain. Immuno-fluorescent microscopy can be used to identify P. multocida in tissue or exudate (Marshall, J.D. 1963).
Bone marrow, heart blood, liver, meninges, or localized lesions are preferred for culturing. To isolate P. multocida, sear the tissue or exudate with a spatula and obtain a specimen by inserting a sterile cotton swab or wire loop through the seared surface. If birds are living, squeeze mucus from the nostril or insert a cotton swab into the nasal cleft. Transfer the specimen to peptone broth and streak on dextrose starch agar containing 5% chicken serum or other suitable media. Specimens also may be streaked on MacConkey and blood agar media to aid in identification.
Colonies characteristic of P. multocida are transferred to dextrose starch agar slants incubated 18–24 hours. Tubes of phenol red broth base containing 1% glucose, lactose, sucrose, mannitol, and maltose, respectively, are then inoculated with growth from the slant. Fermentation of glucose, sucrose, and mannitol without gas is characteristic of P. multocida. Lactose usually is not fermented, but some avian isolates will ferment it. Inoculate 2% tryptose in 0.85% saline solution, incubate 24 hours at 37oC, and test for indole (Kovac’s test). Indole is almost always produced by P. multocida. There should be no hemolysis of blood and no growth on MacConkey agar.
Inoculation of animals may be used as an aid in isolating P. multocida from contaminated materials. Rabbits, hamsters, or mice are inoculated subcutaneously or intraperitoneally with 0.2ml exudate or minced tissue. If P. multocida is present, the animal usually dies within 24–48 hours, and the organism can be isolated in pure culture from heart, blood, or liver.
Serologic diagnosis of FC by rapid whole-blood agglutination, serum plate agglutination, agar diffusion tests, or ELISA has limited value in chronic cholera and no value with the acute form of the disease (David E. Swayne, 2019).
9.2 Differential diagnosis
Avibacterium gallinarum and Gallibacterium anatis biovar haemolytica are 2 closely related bacteria that may be isolated from diseased poultry and incorrectly identified as P. multocida. Avibacterium gallinarum was first described by Hall et al. (1955), who isolated it along with P. multocida from chickens with other maladies characterized by inflammation of the upper respiratory tract. The gel diffusion precipitin test shows a common antigen between Avibacterium gallinarum and P. multocida. Clark and Godfrey (1960) found Avibacterium gallinarum associated with a respiratory disease complex of chickens in southern California. Gilchrist (1963), in a survey of avian respiratory diseases in New South Wales, reported finding Avibacterium gallinarum, Gallibacterium anatis biovar haemolytica, and P. multocida. Harbourne (1962) isolated Gallibacterium anatis biovar haemolytica on 4 occasions from livers of young chickens and turkeys. Gallibacterium anatis biovar haemolytica was isolated from young chickens with salpingitis, which was often accompanied by nasal catarrh, helminth infection, or leukosis; the organism was also isolated from the lungs of fowl with chronic respiratory disease and infectious bronchitis. Matthes et al. (1969) isolated Gallibacterium anatis biovar haemolytica from chickens with a septicemia. Chloramphenicol was effective in treatment. Hacking and Pettit (1974) reported on 8 cases of Gallibacterium anatis biovar haemolytica in pullets and laying hens: 5 cases involved decreased egg production, with some birds showing peritonitis or salpingitis; 3 cases involved increased mortality; some birds had enteritis, enteritis, and hepatitis, or respiratory infection. In most cases, Gallibacterium anatis biovar haemolytica was thought to be a secondary pathogen (David E. Swayne, 2013).
10. Treatment
Antibacterial chemotherapy has been used extensively in the treatment of FC with varying success, depending to a large extent on the promptness of treatment and drug used. Sensitivity testing is often advantageous, because strains of P. multocida vary in susceptibility to chemotherapeutic agents (Donahue, J.M., and L.O. Olson. 1972; Walser, M.M., and R.B. Davis. 1975), and resistance to treatment may develop, especially during prolonged use of these agents.
10.1 Sulfonamides
Several of the sulfonamides have been employed both experimentally and in naturally occurring outbreaks. The main disadvantages of the sulfonamides are their bacteriostatic instead of bactericidal action, inability to cure localized abscesses, and their toxic effect on birds. The use of sulfonamides for treatment of FC has been reviewed (David E. Swayne, 2013).
10.2 Antibiotics
Streptomycin given intramuscularly (IM) at a dose of 150,000mg prevented deaths in adult turkeys when administered before or at the time of inoculation of P. multocida. When treatment was delayed for 6–24 hours or dosage was reduced, chronic infection resulted (McNeil, E., and W.R. Hinshaw. 1948). Penicillin, streptomycin, penicillin and streptomycin, and oxytetracycline (administered IM at the time of experimental exposure of chickens) all possessed activity as therapeutic agents (Bierer, B.W. 1962). Chlortetracycline reduced losses in chicks about 80% when given at the rate of 40mg/kg body weight IM 30 minutes after parenteral inoculation of the organism (Little, P.A. 1948). Chicks that received mash containing 1mg/g had 50% fewer losses than untreated controls. In an outbreak of FC in pheasants, however, Alberts and Graham (1951) did not observe any beneficial results when 1mg/g mash was fed. When chlortetracycline was given IM, a slight reduction in mortality was recorded. Novobiocin administered in feed or water reduced death losses in experimentally exposed turkeys (Hamdy, A.H., and C.J. Blanchard. 1970).
Chloramphenicol (20mg/kg body weight) in a single IM injection was effective in treating FC, but in flocks in which FC and fowl typhoid or fowlpox were concurrently present, chloramphenicol treatment was not successful (Horvath et al., 1962). A chloramphenicol-dexamethasone-pyribenzamine combination was used successfully with vaccination in treatment of FC in breeding turkeys. Respiratory problems, which occurred 1 week after the initial outbreak, responded readily to IM administration of this drug combination (Grant et al., 1968). Water-soluble erythromycin at the rate of 1lb/50 gallon of drinking water halted mortality in two flocks of Muscovy ducklings infected with P. multocida (Hart, L. 1963). Fluoroquinolones are used successfully to treat FC. Pasteurella multocida isolates from poultry are typically highly susceptible to fluoroquinolones (Glisson, J.R. 1995).
Antibiotics used in rations at very low levels for promotion of growth, according to the experiments of Dorsey and Harshfield (1959), did not significantly influence the course of FC infection in inoculated birds. At therapeutic levels, birds that received penicillin and streptomycin in feed died at about the same rate as controls. No deaths occurred in groups that received sulfaquinoxaline or sulfamerazine. These workers also found oxytetracycline and chlortetracycline effective in preventing mortality in experimental FC in a small flock of laying birds; mortality was 80% in an untreated group compared with 12% in a group receiving mash containing oxytetracycline at the level of 500g/ton. In 6 naturally occurring outbreaks, oxytetracycline at this level in feed checked mortality, but losses returned in 3 flocks after withdrawal of the antibiotic.
THE LIST OF PRODUCT IS RECOMMENDED BY VEMEDIM TO TREAT FOWL CHOLERA IN POULTRY.
No. | NAME OF PRODUCT | INGREDIENTS | IMAGE |
1 | Vimequine | Flumequine 100 mg
| |
2 | Tylofos | Fosfomycin 200 mg Tylosin tartrate 50 mg
| |
3 | Trixin | Sulfadimethoxine 250 mg Trimethoprim 50 mg
| |
4 | Tylenro 5+5 | Tylosin 25 mg Apramycin sulfate 30 mg
| |
5 | Trimesul 480 | Sulfadimidin 150 mg Trimethoprim 30 mg
| |
6 | Vime-Cipam | Gentamycin 40 mg Ampicillin Sodium 30 mg
| |
7 | Vimedox 500 | Doxycycline hyclate 550 mg Benzoic acid sodium salt 4 mg
| |
8 | Marbovitryl 250 | Marbofloxacin 25 mg Phenylcarbinol 10 mg Disodium ethylenediaminetetraacetate 1 mg Propylen glycol qs 1 ml
| |
9 | Vime CTC | Neomycin 150 mg Sulfachloropyridazine 100 mg Atropin Sulfate 0.5 mg
| |
10 | Vime Cepcin Fort | Cephaxin 40mg Colistin 180 000 IU Vitamin A 1000 IU Vitamin D3 120 IU Vitamin E 0.5 mg Vitamin B1 2mg Vitamin B6 1mg Vitamin C 3mg |
11. Control and prevention
11.1 Management procedures
Prevention of FC can be effected by eliminating reservoirs of P. multocida or by preventing their access to poultry flocks. Good management practices, with emphasis on sanitation, are the best means of preventing FC. Unlike many bacterial diseases, FC is not a disease of the hatchery. Therefore, infection occurs after birds are in the hands of the producer, and consideration must be given to the many ways that infection might be introduced into a flock.
The primary source of infection is usually sick birds or those that have recovered and still carry the causative organism. Only young birds should be introduced as new stock; they should be raised in a clean environment completely isolated from other birds. Isolation should be extended to housing. Unless separate houses can be provided for first-and second-year layer flocks, the older flock should be marketed in its entirety. Different species of birds should not be raised on the same premises. The danger of mixing birds from different flocks cannot be overemphasized. Farm animals (particularly pigs, dogs, and cats) should not have access to the poultry area. Water fountains should be self-cleaning, and feeders should be covered to prevent contamination as much as possible.
P. multocida has been recovered from many species of free-flying birds and warrants consideration as source of bacteria to poultry; wit measures should be taken to prevent their association with the flock. Raising turkeys in areas where FC is a serious problem may warrant their confinement in houses from which free-flying birds, rodents, and other animals can be excluded. If an outbreak of FC occurs, the flock should be quarantined and disposed of as soon as economically feasible. All housing and equipment should be cleaned and disinfected before repopulation (David E. Swayne, 2019).
11.2 Vaccination
Vaccination should be considered in areas where FC is prevalent, but it should not be substituted for good sanitary practice.
Types of vaccines
Inactivated vaccine: Commercially produced bacterins are available and usually contain whole cells of Heddleston serovars 1, 3, 4 and 3x4 emulsified in an oil adjuvant. The limits of bacterins are that the protection is limited to strains that share a highly similar or identical LPS structure. Because the determination of LPS structures is highly specialized, it is not possible to confirm that a field isolate will be covered by a bacterin, even if the field isolate is identified and shown to be a serovar that matches the bacterin content. Hence, autogenous bacterins containing a locally isolated strain other than serotypes 1, 3, or 4 may be used, although the results are often mixed. The mixed results with autogenous bacterins may be cause by the presence of multiple genotypes and serovars as has been recently shown in ducks, turkeys and layers. Also, the need for a close match in LPS structure may explain the mixed results.
The choice of adjuvant for a bacterin (commercial or autogenous) can be water‐in‐oil emulsion or aluminum hydroxide. Bacterins using aluminum hydroxide as the adjuvant are useful for the vaccination of turkey breeder or broiler breeder flocks that are in lay because the water‐ in‐oil emulsion, in combination with the whole bacterial cell, results in a significant tissue response by the bird. This response can result in significant declines in egg production. The negative effect on egg production is less with aluminum hydroxide adjuvant whole‐cell FC bacterins. It has been well documented that aluminum hydroxide bacterins do not stimulate the immune response as well as water‐in‐oil bacterins. Therefore, if an aluminum hydroxide bacterin is used, revaccination may be required to afford immunity to a flock for an entire laying cycle.
Live vaccine: Three live vaccines available for use in the United States are CU, a strain of low virulence; M-9, a mutant of CU with very low virulence; and PM-1, a mutant of CU intermediate in virulence between CU and M-9. Vaccination of chickens and turkeys with these live P. multocida vaccines induces protection against heterologous serotype challenge. The use of live FC vaccines stimulates an effective immune response but has the disadvantage of potentially resulting in mortality in the vaccinated birds. If the mortality post vaccination becomes excessive, it can be reduced by the administration of an antibiotic. This should be avoided, if possible, until at least 4 days post vaccination when there will be at least partial immunity induced by the vaccine (David E. Swayne, 2019).
Field vaccination protocols and regime
When considering the most appropriate vaccination program for FC, the following should be taken into consideration: prevalence of FC in the area, most prevalent serotypes of P. multocida in area, age of birds to be vaccinated, and the value of the birds to be vaccinated (i.e., breeder turkeys vs. commercial turkeys or parent chicken breeders vs. grandparent chicken breeders). There have been many successful vaccination protocols for chicken breeders against FC. Bacterins, live vaccines, or both are used, and usually 2 doses are given: the first at 8–10 weeks of age and the second at 18–20 weeks of age. Protection occurs only against serotypes contained in the bacterin and does not give solid immunity for an entire laying cycle. Some of the more commonly used vaccination programs consist of administering a live vaccine in the wing web at 10–12 weeks of age followed by either another live vaccine in the wing web or a bacterin at 18–20 weeks. Vaccination with live vaccine provides protection against multiple serotypes, but the vaccine can cause chronic FC. The use of a bacterin at 10–12 weeks and a live vaccine at 18–20 weeks, just prior to movement to the laying house, gives protection against multiple serotypes and minimizes live vaccine-induced chronic FC.
One of the most successful programs for vaccination of both breeder turkeys and commercial meat turkeys is the use of a live vaccine in the drinking water every 4 weeks beginning at 6–8 weeks of age and continuing for the life of the flock. Bacterins also can be used in breeder turkeys. They are vaccinated 2–5 times before the onset of egg production, with the first vaccination beginning at 6–8 weeks (David E. Swayne, 2019).
12. References
Boulianne, M., Blackall, P.J., Hofacre, C.L., Ruiz, J.A., Sandhu, T.S., Hafez, H.M., Chin, R.P., Register, K.B., Jackwood, M.W., 2020. Pasteurellosis and Other Respiratory Bacterial Infections, Diseases of Poultry, pp. 831-889.
Heddleston, K.L. and Wessman, G., 1975. Characteristics of Pasteurella multocida of human origin. Journal of clinical microbiology, 1(4), pp.377-383.
Hungerford, T.G. 1968. A clinical note on avian cholera. The effect of age on the susceptibility of fowls. Aust Vet J. 44:31–32.
J. P. Christensen and M. Bisgaard. 1997. Avian pasteurellosis: Taxonomy of the organisms involved and aspects of pathogenesis, Avian Pathology, 26:3, 461-483.
Parın Uğur, Kirkan Sukru and Erbas Goksel. 2018. Emerging bacterial zoonoses in migratory birds.
Peng, Zhong, Xiangru Wang, Rui Zhou, Huanchun Chen, Brenda A. Wilson, and Bin Wu. 2019. Pasteurella multocida: Genotypes and Genomics, Microbiology and Molecular Biology Reviews, 83: e00014-19.
Pasteur L. 1880. De l’attenuation du virus du cholera des poules. C R Acad Sci 91: 673–680.
Pasteur, L. 1880a. Sur les maladies virulents et en particulier sur la maladie appelee vulgairement cholera des poules. CR Acad Sci. 90:239–248, 1030–1033.
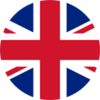