PLEUROPNEUMONIA OF SWINE
Nguyen Pham Thao Nhi, Tran Duy Thanh, Pham Trang Thanh Nguyen,
Nguyen Khanh Thuan, Nguyen Phuc Khanh, Nguyen Thanh Lam*
1. Introduction
Actinobacillus pleuropneumoniae (APP) (originally named as Haemophilus pleuropneumoniae) is the etiologic agent of porcine pleuropneumonia. An organism also isolated from porcine pleuropneumonia, originally known as “Pasteurella haemolytica‐like” was later determined to be a nicotinamide adenine dinucleotide independent biotype of A. pleuropneumoniae and is known as A. pleuropneumoniae biotype II.
A. pleuropneumoniae is one of the most important swine bacterial respiratory pathogens and is found worldwide. The most virulent strains can induce rapidly fatal fibrinohemorrhagic and necrotizing pleuropneumonia in swine of all ages. Survivors often have devitalized bacterialaden sequestra in their lungs. The economic importance of A. pleuropneumoniae is mainly due to the mortality, reduced growth, veterinary costs (antimicrobials, vaccinations), and condemnations at the slaughterhouse. However, in chronically infected herds, results from studies investigating impact on average daily gain have been controversial.
Virulence of A. pleuropneumoniae strains varies remarkably; some strains produce high mortality, others are avirulent, and yet others are intermediate in virulence. High mortality outbreaks are now rather infrequent in the United States and Canada, but remain a problem in Latin American, Asian, and European countries. Certain A. pleuropneumoniae strains can also act as secondary pathogens contributing to increased mortality in conjunction with other viral and bacterial pathogens as a component of the porcine respiratory disease complex (PRDC).
A. pleuropneumoniae infections can be frustrating for swine producers and veterinarians. Many herds are infected with several strains. High virulence strains may be present in a herd for extended periods of time without evidence of clinical signs or even lesions at the slaughterhouse. The organism resides mainly in the tonsils, and carrier pigs can be difficult to detect. Outbreaks may suddenly appear in the presence of concomitant diseases or concurrent with changes in management or other significant stressors such as overcrowding. There are not readily available laboratory tests to differentiate highly virulent strains from strains of lesser virulence.
Both the organism and the disease have been studied extensively. Knowledge gained has allowed for better diagnostic tests and strategies, vaccines, and relatively effective eradication strategies. However, A. pleuropneumoniae remains a significant cause of economic loss to the swine industry, and there remains opportunity for improvement in control and eradication of this agent (Gottschalk and Taylor, 2006).
2. Aetiology
2.1 Bacteria characteristics
Pleuropneumonia is a severe respiratory infection caused by A. pleuropneumoniae (APP). A. pleuropneumoniae, previously designated as Haemophilus pleuropneumoniae or H. parahaemolyticus, member of the family Pasteurellaceae, genus Actinobacillus, species pleuropneumoniae, is the cause of pleuropneumonia of swine.
A. pleuropneumoniae is a small, gram‐negative encapsulated rod with typical coccobacillary morphology. A. pleuropneumoniae forms colonies 0.5–1 mm after 24 hours of incubation and is usually beta‐hemolytic. In fact, A. pleuropneumoniae produces an increased zone of hemolysis within the zone of partial lysis surrounding a beta‐toxigenic Staphylococcus aureus or Staphylococcus epidermidis (the CAMP phenomenon). This CAMP phenomenon is due to secretion of combinations of the cytolysins ApxI, ApxII, and ApxIII by various strains of A. pleuropneumoniae. Additional detailed morphologic and biochemical characteristics may be found in original reports. Biotype I strains should be differentiated from other NAD‐ dependent actinobacilli present in the upper respiratory. tract of swine such as A. minor, A. porcinus, or A. porcitonsillarum. Definitive identification may require species‐specific polymerase chain reaction (PCR) testing. Biotype II strains grow easily on blood agar plates without the presence of NAD; colonies must be differenced from those of A. suis using a panel of biochemical tests or by PCR. This is especially important because A. suis may induce pleuropneumonia similar to A. pleuropneumoniae but has different implications for control.
A. pleuropneumoniae biotype I has been divided into 14 serotypes (1–12, 15, and 16) and biotype II into 2 serotypes (13–14) for a total of 16 serotypes. Serotype 5 is subdivided into subtypes 5a and 5b; however, this subdivision has neither epidemiological nor pathological significance, and most laboratories do not perform the subtyping. The above association of serotypes and biotypes is predominant but not exclusive. Biotype II strains belonging to serotypes 2, 4, 7, 9, and 11 (normally found among biotype I strains) have been reported. Additionally, biotype I serotype 13 strains that are antigenically different from the reference serotype 13 biotype II strain have been isolated from the United States and Canada. Untypable biotype I or biotype II strains are sometimes isolated in both North America and Europe, and new serotypes will probably be described.
Serotype specificity is considered to be conferred by the capsular polysaccharides (CPS). CPS are usually associated with particular cell wall lipopolysaccharides (LPS), i.e. CPS type 1 is usually associated with LPS type 1. However, some capsular serotype groups share identical O‐chain LPS, thus explaining the cross‐reactions observed in serological assays using LPS as antigen between serotypes 1, 9, and 11; serotypes 3, 6, 8, and 15; and serotypes 4 and 7. Yet, isolates with a given capsular type and a different LPS type have been sporadically reported. For example, strains of CPS/LPS serotype 1/7 and 2/7 have been reported in North America and Europe, respectively whereas a CPS/LPS 12/3 strain has been recently described in Japan. The biotype I serotype 13 strains recovered in North America are antigenically different from the biotype II serotype 13 reference strain (isolated in Europe) in that they have an LPS that cross‐reacts with that from
A. pleuropneumoniae
serotype 10. These strains can cause false‐positive serotype 10 serologic reactions in LPS‐based serologic tests. It has been suggested that serotypes of
A. pleuropneumoniae
should be more rigorously defined by specifying both capsular (K) and LPS (O) antigens, but this nomenclature has not been widely adopted (Gottschalk and Taylor, 2006).
2.2 Classification
2.3 Pathogenicity
Outbreaks of disease caused by A. pleuropneumoniae are usually associated with intensive production of pigs. In conditions of high stock density, rapid growth, poor ventilation etc., the disease can spread rapidly among animals with no immunity. Many animals can die in such an outbreak, while others will recover only partially, having residual lesions in the lung. Scarring can predispose to future infections with other bacteria, and pleural adhesions may inhibit normal respiratory function and cause poor growth. As herd immunity among survivors increases, the acute disease becomes less common, but animals continue to harbour the agent. Colostral antibody derived from infection in the sow is transferred to her piglets (Nielsen, 1975). It was also reported that protection lasts for no longer than 3 weeks, although antibody may persist for several weeks, during which time piglets are exposed to the organism and active immunity develops at this time. Therefore, further outbreaks of infection in a herd tend to occur in groups of animals where insufficient immunity is present (Nielsen and Mandrup, 1977).
The acute disease was systematically studied by it is a necrotizing, fibrohaemorrhagic pneumonia with pleurisy. There is severe congestion in the lung and haemorrhage and exudation of serosanguinous fluid into the pulmonary parenchyma. Sites of bacterial growth are rapidly infiltrated with neutrophils which then rapidly degenerate. Septicaemia is a rare, usually terminal complication of the infection. For many years, the primary mediator of damage was considered to be endotoxin. While a role for endotoxin has not been excluded, and indeed the endotoxin of A. pleuropneumoniae is considered to be unusually puissant, intensive research has revealed a primary role for the protein Apx toxins in the invasive ability and lesion production by this organism (Bertram, 1990).
A. pleuropneumoniae has always been known to be haemolytic, as visualized by the CAMP effect. Indeed, this remains one dependable means of recognizing the organism. In 1980, Soren Rosendal and colleagues in Guelph published work reporting the discovery that lung lesions could be induced in pigs using cell-free culture fluid. They followed this with a landmark paper describing how heat-labile, cell-associated and heat-stable, cell-free extracts of a virulent isolate of H. pleuropneumoniae were toxic to porcine cells, particularly alveolar macrophages. This, together with the rapidly increasing problem of pleuropneumonia in growing pigs, was a potent stimulus for further examination of the causal agent. Investigations of the nature and role of the haemolysin then began. Early suggestions that the haemolysin may be involved in the disease, who reported a heat-stable haemolytic activity in H. pleuropneumoniae. They expanded upon this to reveal that the haemolytic substance was also cytotoxic for porcine macrophages, and that it was carbohydrate in nature. Another group reported the haemolysin to be related to the group of toxins typified by Streptolysin S and the haemolysin of Serpulina hyodysenteriae. They found that haemolytic activity was protease-sensitive and heat-labile, but was extremely variable and unreliable, and appeared to depend on the presence of RNA as a carrier molecule. However, only certain batches of RNA were effective and, in hindsight, this may have been due to unrecognized contamination with calcium ions required for haemolytic activity by the Apx toxins. Similarly, reported a heat-labile haemolysin detectable in cultures from H. pleuropneumoniae serotype 3. Crucially, they included 10 mM calcium ions in their assay buffer, and the results they obtained were more consistent.
To rationalize the disparate nomenclature which had arisen through different laboratories’ use of different names and gene designations, it was proposed to re-designate the haemolysin and cytotoxin molecules of A. pleuropneumoniae ApxI, ApxII and ApxIII. The work of many laboratories towards the understanding of these toxins was then brought successfully together (Rycroft and Garside, 2000).
Table 1.
Examples of gap(s) identified and hypotheses formulated from misteachings analysis (Langford et al., 2021)
Category | Example gap(s) identified | Example hypothesis |
Microbiome | Lack of knowledge on whether the lung and/or gut and/or tonsil microbiome can reduce susceptibility of pigs to A. pleuropneumoniae infection | That the prior presence of specific bacterial species or microbiota can prevent colonization of the tonsils by A. pleuropneumoniae That specific species present in the gut microbiota enhance A. pleuropneumoniae vaccine efficacy through cross talk with the lung microbiota |
Immunity | Lack of information on Th17 responses to A. pleuropneumoniae during infection and their role in immunity Lack of information on mechanisms of disease tolerance that are involved in immunity to A. pleuropneumoniae | That rationally designed vaccines targeting the Th17 response can be cross-protective against all serovars That immunomodulators targeting disease tolerance mechanisms can prevent A. pleuropneumoniae disease |
Sex | Lack of information on sex-dependent differences in immune response to A. pleuropneumoniae | That A. pleuropneumoniae vaccines can be rationally designed to prevent tonsillar colonization of sows |
Temperature | Lack of information on the role of temperature as a regulator of A. pleuropneumoniae virulence gene expression | That the pig body temperature is a major regulator of A. pleuropneumoniae virulence factor expression |
Environment | Lack of algorithms based on environmental parameters predicting the likelihood of A. pleuropneumoniae disease in a unit Lack of controlled studies investigating the effect of environment on susceptibility of pigs to A. pleuropneumoniae | That simple algorithms can be formulated predicting the likelihood of A. pleuropneumoniae disease in a unit |
Chance | Lack of information on the long range transmission of A. pleuropneumoniae | That transmission is possible over long distances (>500 m) between farms |
Inoculum | Lack of information on the bacterial phenotype in the environment | That A. pleuropneumoniae grown in biofilms in the environment are less transmissible than those in aerosols created by coughing |
Nutrition | Lack of information on the specific (non-antimicrobial) dietary supplements that would decrease A. pleuropneumoniae susceptibility | That insect-based dietary supplements would enhance immunity and decrease A. pleuropneumoniae susceptibility to disease |
Genetics | Lack of information on both A. pleuropneumoniae and host−cell interactive ligands | That deletion of the tonsillar epithelial cell receptor in pigs will lead to A. pleuropneumoniae-colonization resistant pigs |
Strain | That there are only a small number of publicly available whole genome sequences | That specific A. pleuropneumoniae genes are essential for co-infection with other pathogens of the PRDC |
3. History
In the United States, A. pleuropneumoniae was first reported in 1957. It was reported frequently in the 1960s and 1970s. Frequency of outbreaks increased with the industrialization of swine production. Research has been greatly facilitated by use of infected seeder pigs to reproduce A. pleuropneumoniae under experimental conditions. Because of the economic consequences of disease, most producers strive to eliminate infection from herds (https://vetmed.iastate.edu).
4. Epidemiology
4.1 Geographic distribution
A. pleuropneumoniae is widely distributed and only infects pigs, although a recent report suggested that an A. pleuropneumoniae strain was able to infect poultry. The primary reservoir is domestic swine, but various studies in wild boar populations in Europe, North America, and Australia showed that this species is frequently infected by A. pleuropneumoniae. In Canada, these populations are serologically positive for serotype 14, a capsular type not found so far in domestic pigs in North America.
Outbreaks of pleuropneumonia have been reported in domestic swine from practically all European countriesand from different parts of the United States, Mexico, South America, Japan, Korea, Taiwan, and Australia as well as in many other countries. Interestingly, there has been a simultaneous increase in clinical A. pleuropneumoniae in recent years in many European countries as reflected in increased chronic pleuritic adhesions in slaughterhouses. This is possibly due to older weaning ages mandated by new welfare laws, since A. pleuropneumoniae is known to be a late colonizer in piglets and earlier weaning dramatically reduces carriage rates in weaned pigs.
The distribution of serotypes involved in acute out breaks and chronic infections in different regions of the world is radically different. Moreover, strains of a given serotype may be highly virulent in one region, and yet strains of the same serotype may be of low virulence in another region. Therefore, it is critical when testing imported pigs for A. pleuropneumoniae prior to introduction into a closed herd to select diagnostic tests for the most important virulent serotypes present in their region of origin.
Unfortunately, only a few reports on the distribution of serotypes recovered from diseased animals have beenpublished during the last 25 years. Moreover, the relative prevalence of serotypes may dramatically change during the years as described in Korea and Canada. In some regions, one or more virulent serotypes predominate and cause most outbreaks serotypes5 and 7 in North America, serotypes 2 and 9 in many European and Asian countries, and serotype 15 in Australia, to name a few. Serotype 2 strains are highly virulent in European and Asian countries owing to secretion of two cytotoxins, ApxII and ApxIII, but serotype 2 strains in North America secrete only ApxII and are oflow virulence. Serotype 4 is one of the most frequent virulent serotypes in Spain, but isuncommon in most other countries. This serotype was isolated once from asymptomatic animals in Canada, but there are no other reports of serotype 4 strains in NorthAmerica. Serotype 15, originally described and the predominant serotype in Australia, has also occasionally been reported in North and South America as well as in Japan. Serotype 8 is by far the predominant serotype in theUnited Kingdom and occasionally isolated from diseasedpigs in North America and Japan.
Biotype II strains are more commonly isolated in some European countries; however, these strains are occasionally reported in North America. Biotype II strains have traditionally been considered of low virulence; however, there are reports of their isolation in several cases of fatal pleuropneumonia. Reported that 25% of isolates recovered from diseased pigs in Spain belonged to biotype II of whichserotypes 7, 2, 4, and 11 were the most common.
Most conventional herds are infected with one or more serotypes of A. pleuropneumoniae, and although these strains are often of low virulence, high virulence strains may also be present. Most prevalent serotypes (detected by serology) are often different from thoserecovered from diseased pigs. In a Spanish report, almost 90% of studied herds were serologically positive for A. pleuropneumoniae, many of them presenting pleuritic lesions at slaughterhouse, although the distribution of involved serotypes was not studied. In a Canadian study, 78% of herds were A. pleuropneumoniae positive based on PCR detection in the upper respiratory tract of piglets. In the same study, 70% of the herds were seropositive by LPS‐ELISA with distribution of serotypes as follows: 26% were positive for serotype 7/4, 17% for 12, 15% for 3/6/8/15, 6% for 5, 4% for 2 and 2% for 1/9/11. Although serotypes 2, 12, 3, 6, 8, and 15 are usually considered as of low/intermediate virulence, serotypes 7 and 5 are currently the most common serotypes recovered from diseased pigs in Canada. Interestingly, most herds included inthe study did not present clinical signs of pleuropneumonia. The somewhat paradoxical trend of high seroprevalence combined with low disease prevalence highlights a concept important in the epidemiology of A. pleuropneumoniae. Low virulence strains tend to be highly prevalent in herds, resulting in high seroprevalence; however, highly virulent strains are carried by a much lower proportion of animals, resulting in lower seroprevalence. This has to be taken into account when determining the number of animals to be tested in serological surveillance programs.
Strains belonging to the same serotype have been genotypically compared. Although a given genotype profile cannot be clearly related to a given serotype, several studies have shown a relative homogeneity within a particular serotype with one or few clones represented and this, depending on the technique used. Further rapid subtyping methods are needed, and a combination of different methods will have to be used to reach conclusions about epidemiological relationships. Complete genome sequencing has also been used to compare different strains belonging to the same serotype (Gottschalk and Taylor, 2006).
Table 2.
Geographic distribution of A. pleuropneumoniae serotypes in the world(Dubreuil et al., 2000).
Country | Prevalent serotypes | Dominant serotype(s) |
Argentina | 1, 2, 3, 5, 12 | 1 |
Australia | 1, 2, 3, 7, 12 | 1 |
Belgium | 2, 3, 6, 7, 8, 9, 11 | 3 |
Brazil | 1, 3, 4, 5, 7, 9 | 5, 3 |
Canada | 1, 2, 3, 5, 6, 7, 8, 10 | 5, 7, 1, 12 |
Chile | 1, 5 | 1, 5 |
Croatia | 2, 7, 8, 9 | 2, 9 |
Czechoslovakia | 1, 2, 7 | 2 |
Denmark | 1, 2, 3, 5, 6, 7, 8, 10, 11, 12 | 2 |
France | 2, 3, 7, 8, 9 | 9 |
Germany | 2, 3, 4, 5, 6, 7, 9, 10 | 9, 2, 7 |
Hungary | 1, 2, 3, 5, 6, 7, 9, 10, 11, 12 | 3, 2, 7 |
Italy | 1, 2, 3, 4, 5, 7 | 5 |
Ireland | 3 | 3 |
Japan | 1, 2, 3, 5, 6, 7, 8, 9, 12 | 1, 2 |
Korea | 2, 3, 5, 7 | 5, 2 |
Mexico | 1, 2, 3, 4, 5, 6, 7, 8, 9 | 1, 8 |
Netherlands | 1, 2, 3, 5, 7, 8, 9, 11 | 2, 9, 11 |
Norway | 2 | 2 |
Poland | 1, 2, 5, 9 | 1, 9 |
Spain | 1, 2, 3, 4, 5, 6, 7, 8, 9, 10, 12 | 4, 7, 2 |
Sweden | 2, 3, 4 | 2 |
Switzerland | 2, 3, 7, 9 | 2 |
Taiwan | 1, 2, 3, 5 | 1, 5 |
UK | 1, 2, 3, 5, 6, 7, 8, 10 | 2, 3, 8 |
USA | 1, 3, 5, 7, 8, 9 | 1, 5 |
Venezuela | 1, 7, 4, 2 , 3, 6 | 1 |
4.2 Transmission
With few exceptions of systemic, A. pleuropneumoniae is essentially a swine respiratory pathogen. In peracute and acute infections, it can be found not only in pneumonic lung but also in large numbers in nasal discharges. Survivors may remain carriers for several months mainly in chronic lung lesions and tonsils. Some exposed animals remain healthy and become subclinical carriers mainly in the tonsils. Less frequently, carrier animals may harbor the organism in the nasal cavity. Subclinical carriage of A. pleuropneumoniae in tonsils occurs not only for low virulence strains but also for high virulence strains that can sometimes be present in well‐managed herds for long periods without clinical signs or lesions. Environmental stressors or concurrent respiratory pathogens can result in sudden clinical outbreaks. Transmission between herds occurs mainly through the introduction of carrier animals. The main route of spread is by direct nose‐to‐nose contact or by droplets within short distances. Moving and mixing pigs increases the risk of spread. In acute outbreaks, infection may not occur in every pen, suggesting the possible role of aerosols and air movement in the transmission of the disease over longer distances within buildings, or the indirect transmission of contaminated exudates from acutely infected pigs by farm personnel. Studies confirm that A. pleuropneumoniae may be transmitted by aerosol over short distances. Kristensen et al., 2004 reported that airborne transmission between closely located pig units is possible, but uncommon. In contrast, Zhuang et al., 2007 suggested that local spread of A. pleuropneumoniae serotype 2 from neighboring herds was a predominant factor in the contamination of Danish specific‐pathogen‐free (SPF) genetic herds. Introduction of the disease by artificial insemination or embryo transfer is highly unlikely, since the genital tract is not a common site of infection and antimicrobials in the semen diluent or the embryo washing procedures could possibly prevent the persistence of the organism. Birds and small rodents are unlikely to transfer A. pleuropneumoniae and are not considered significant sources of the infection. The role of A. pleuropneumoniae‐contaminated fomites in the transmission of the infection is suspected but has not been studied.
In endemically infected herds, A. pleuropneumoniae is transmitted by infected sows to their offspring via close contact. The frequency of transmission is likely related to the amount of bacteria shed by the sow, although more quantitative studies are needed. A recent study showed that although most sows were colonized, only some litters of piglets (and some piglets within a litter) were infected. It has been suggested that the level of antitoxin maternal antibodies does not influence the colonization of piglets. The level of maternal antibodies against the LPS, however, seems to be positively correlated with a late colonization. The persistence of colostral antibody in piglets ranges from 2 weeks to 2 months of age, depending on the initial level of acquired colostral antibodies, the antigen used in the test, and the serological test used. Usually only a few piglets are infected from their dams during the late suckling period; then lateral spread occurs after weaning as decay of maternal immunity renders more pigs susceptible or if mixed with native pigs.
Survival of the organism in the environment is of short duration, especially in warm, dry conditions. However, when protected with mucus or other organic matter, it can survive for several days (even weeks), and it can survive in clean water for periods of up to 30 days at 4oC). Common disinfectants are effective against A. pleuropneumoniae when organic matter is first removed by thorough washing (Gottschalk and Taylor, 2006).
5. Pathogenesis
Virulence factors and pathogenesis of the infection
The incubation period can be quite variable. Inoculation of pigs with large numbers of virulent A. pleuropneumoniae can lead to fatal pleuropneumonia in as few as 6 hours. Following exposure by oronasal contact or inhalation, A. pleuropneumoniaefirst colonizes the epithelial cells on the surface and later in the crypts of the palatine tonsil. Colonized epithelial cells vacuolate and desquamate and, along with transmigrating neutrophils, distend tonsillar crypts. In contrast, A. pleuropneumoniae does not bind well to the ciliated epithelium of the trachea or bronchi; an exception may be the trachea of newborns. When able to reach the lower respiratory tract, A. pleuropneumoniae is able to adhere to pneumocytes that linealveoli.
Colonization is dependent upon bacterial‐to‐cell adhesion that seems to be mediated by polysaccharides and proteins. Evidence from other pathogen host cell interactions suggests that adherence to host cells is a complex and multifactorial process. The oligosaccharide core of LPS seems to play an important role in adherence of A. pleuropneumoniae to swine cells. The presence of proteinaceous fimbriae and fimbrial subunits on the surface of A. pleuropneumoniae has been demonstrated. However, their eventual role in adhesion remains to beelucidated.
Once in the respiratory tract environment, certain bacterial nutrients, particularly iron, are scarce. A. pleuropneumoniae expresses a number of factors that are involved in the acquisition and uptake of iron. Among other mechanisms, A. pleuropneumoniae is capable of utilizing porcine transferrin and heme compounds including free heme, hemin, hematin, and hemoglobin as well as a siderophore.
In pigs that are tonsillar carriers of virulent strains of A. pleuropneumoniae, the mucociliary apparatus normally clears any stray bacterial cells that are inhaled. This prevents A. pleuropneumoniae access to and subsequent replication in alveoli, the essential step to development of pleuropneumonia. Factors that overwhelm or reduce the function of the mucociliary apparatus are necessary to deliver sufficient A. pleuropneumoniae to alveoli for subsequent disease development. High inhaled doses of A. pleuropneumoniae in finely atomized particles can result in A. pleuropneumoniaereaching alveoli. This is most likely during acute outbreaks when sick pigs are shedding large amounts of A. pleuropneumoniae. Alternatively, cilia can be damaged by colonizing Mycoplasma hyopneumoniae orby replication of pseudorabies (Aujeszky’s) virus or influenza virus in tracheal and bronchial epithelial cells. Showed that experimental inoculation of 10‐week‐old pigs with a serotype 9 strain of A. pleuropneumoniae produced disease only in pigs previously exposed to M. hyopneumoniae and not in non‐exposed controls. Other factors may also impair ciliary function, like chilling or high environmental ammonia levels. It has been suggested that out breaks of A. pleuropneumoniae on endemic farms could be caused by a trigger inducing pneumonia in already infected pigs.
Once in the alveoli, a battle ensues between host innate and acquired immune mechanisms in the alveoli and virulence mechanisms of A. pleuropneumoniae to overcome them, the outcome determining whether A. pleuropneumoniae is killed or causes pleuropneumonia. Initially LPS on the surface of A. pleuropneumoniae acts a potent attractor of macrophages and neutrophilsas well as stimulates host alveolar macrophages to secrete inflammatory cytokines. It has been recently reported that a local expression of proinflammatory cytokines during A. pleuropneumoniae infection would play an important role in the pathogenesis of swine pleuropneumonia. In fact, these cytokines would activate macrophages and increase vascular permeability, flooding alveoli with important antibacterial serum proteins including complement and anti‐A. pleuropneumoniae IgG antibodies (maternally derived, vaccinated, or previously exposed animals). A. pleuropneumoniae has several strategies to resist these host responses. The polysaccharide bacterial capsule of A. pleuropneumoniae inhibits engulfment by phagocytes as has been demonstrated by the characterization of non‐virulent isogenic non‐capsulated mutants. Both macrophages and neutrophils can phagocytose A. pleuropneumoniae only in the presence of convalescent pig serum owing to the opsonic activity of anti‐A. pleuropneumoniae IgG. A. pleuropneumoniae is also resistant to the action of complement.
The most important factors involved in impairment of the phagocytic function of both macrophages and neutrophils are the secreted protein RTX toxins ApxI, ApxII, and ApxIII. ApxI is strongly hemolytic andcytotoxic, ApxII is weakly hemolytic and moderately cytotoxic, and ApxIII is nonhemolytic but strongly cytotoxic. ApxI toxin has been shown to induce apoptosis in porcine alveolar macrophages, whereas ApxIII is highly toxic for peripheral blood mononuclear cells. In general, strains of serotypes 1, 5, 9, and 11 produce ApxI and ApxII; strains of serotypes 2, 3, 4, 6, 8, and 15 produce ApxII and ApxIII; strains of serotypes 7, 12, and 13 produce only ApxII; and strains of serotypes 10, 14, and 16 produce only ApxI. It seems that strains of serotype 3 secrete low levels of ApxII. A fourth toxin (ApxIV) is produced (in vivo only) by all serotypes. The possible role of this toxin on phagocyte damage remains to be elucidated. Using an ApxIV knockout mutant, it has been reported that ApxIV would be essential for expression of full virulence of A. pleuropneumoniae, although more studies are needed to confirm such hypothesis.
Finally, other virulence factors have also been suggested to play important roles in the pathogenesis of the infection, such as outer membrane proteins, proteases, and many products coded by genes that are clearly upregulated during infection, although in some cases their exact function is not yet known. The capacity of A. pleuropneumoniae to form biofilms with a certain implication during A. pleuropneumoniae chronic infection and/or colonization has also been suggested as being part of the pathogenesis of the infection. Finally, genome sequencing and preliminaryanalysis of strains belonging to serotypes 2, 3, 5, 6, 7, and 8 have been reported. The analysis of the functions of the encoded proteins will extend current knowledge on the metabolic and virulence characteristics of this pathogen.
Tissue damage in the lung is extensive and due principally to the combined effects of the Apx cytotoxins on a variety of lung cells and the A. pleuropneumoniae LPS‐stimulated host inflammatory response. Host phagocytes are attracted by LPS and Apx‐induced chemokines. Macrophages are activated and secrete toxic oxygen metabolites, and macrophages as well as neutrophils are killed by Apx toxins and release lysosomal enzymes, which together further damage lung cells. Damage to endothelial cells results in activation of the coagulation pathway, microthrombus formation, and localized ischemic necrosis. In most fatal casesof peracute pleuropneumonia, death is caused by endotoxic shock arising from absorption of copious amounts of A. pleuropneumoniae‐derived LPS (Gottschalk and Taylor, 2006).
Factors affecting severity of disease
Differences in virulence between serotypes or even within the same serotype have often been observed. In the field, strains of serotypes 1, 5, and 7 in North America and of serotypes 2 and 9/11 (the latter being extremely difficult to be differentiated) in Europe are generally found to be more virulent than those of other serotypes. It is suggested that such differences are due to their combination of Apx toxins/hemolysins, capsular structure, and LPS composition. In addition to production of ApxIV by all strains, virulent strains seem to produce 2 (rather than 1) of either Apx I, II, or III. Interestingly, atypical strains that are either more or less virulent than others in their respective serotype have often been shown to have either added (more virulent) or lost (less virulent) an Apx toxin. However, not all differences in virulence are explained by capsule, LPS, and Apx toxin profiles as exemplified by some low virulence serotype 1 strains that have no atypical CPS, LPS, or toxin profiles. Attempts have been made using molecular genetic techniques directed toward Apx toxin and other gene targets to differentiate degrees of virulence in strains of A. pleuropneumoniae; however, there are currently no methods to definitively differentiate virulence levels of A. pleuropneumoniae strains apart from controlled animal inoculation experiments.
Outcome of infection by A. pleuropneumoniae and severity of outbreaks as determined by increased morbidity and mortality can be impacted by several factors. As already mentioned, strain virulence and the presence of other pathogens like M. hyopneumoniae, pseudorabies virus, and, likely, swine influenza virus significantly impact disease. Interestingly, serotypes considered as low pathogenic (such as serotypes 6, 8, 12, and 15, at least in North America) cansometimes induce necrotizing bronchopneumonia or pleuropneumonia, especially in the presence of these pathogens. In fact, the same strain may either behave as completely avirulent or induce serious clinical disease in the absence or presence of a M. hyopneumoniae coinfection. A synergy between PRRSV and A. pleuropneumoniae is controversial. Early experimental studies showed that a coinfection does not result in more severe A. pleuropneumonia induced disease. This is in agreement with field observations in areas of endemic PRRSV infection (such as certain regions of Canada) where the incidence of clinical pleuropneumonia has not significantlyincreased. Even an antiviral effect of A. pleuropneumoniae antigens has been reported. However, a certain effect of the coinfection has been suggested for acute and chronic (pleuritic) infections. Factors such as crowding and adverse environmental conditions such as rapid changes in temperature and high relative humidity coupled with insufficient ventilation also promote the development and spread of the disease and, consequently, affect morbidity and mortality. It is therefore not surprising that the highest incidence of outbreaks is observed in growing and finishing pigs, mainly in seasons with adverse weather conditions, and in herds where pigs from numerous sources are mixed.
Immune status of the animals relative to the infecting serotype of A. pleuropneumoniae is also important, whether passive, convalescent, or vaccinal. Animals from conventional herds that might have been in contact with low virulent serotypes of A. pleuropneumoniae or with A. suis may be more resistant to the infection from a specific strain than SPF animals that are negative to all serotypes of A. pleuropneumoniae. However, this may depend on the ability of the previous colonizing serotypes to induce antibodies against the toxins produced by the newly infecting strain (Gottschalk and Taylor, 2006).
6. Clinical signs and pathology
Clinical signs vary with the age of the animals, their state of immunity, the environmental conditions, and the degree of exposure to the infectious agent. The clinical course can be peracute, acute, or chronic. All stages of disease – from intermediate to fatal, subacute, or chronic – may develop within an affected group.
In the peracute form, one or more pigs in the same or
different pens suddenly become sick with high fever to 106.7oF (41.5oC), apathy, and anorexia. Some pigs may vomit. The affected animals lie on the floor without distinct respiratory signs, heart rate is increased, and cardiovascular function fails. The skin on the nose, ears, legs, and later the whole body becomes cyanotic. In the terminal phase, there is a severe dyspnea with mouth breathing, animals remain in a sitting posture, and rectal temperatures drop. Shortly before death, there is usually a copious, foamy, blood‑tinged discharge through the mouth and nostrils. In the peracute form, it is also common to find one or more animals dead without any premonitory signs and with typical foamy, blood‑tinged nasal discharge. Experimental studies have shown that the course of the disease may be as little as 6 hours from infection to death (Gottschalk and Taylor, 2006).
In the acute form, many pigs in the same or different pens are affected. Body temperature rises to 105–106oF (40.5–41oC), the skin may be reddened, and the animals are depressed, are reluctant to rise, refuse food, and are reluctant to drink. Severe respiratory clinical signs with dyspnea, cough, and sometimes mouth breathing are evident. The course of the disease differs from animal to animal, depending on the extent of the lung lesions and the time of initiation of therapy.
The chronic form develops after the disappearance of acute signs or when treatment or preventive measures are not able to completely control the infection and/or
infections due to intermediate virulence serotypes. There is little or no fever, and a spontaneous or intermittent cough of varying intensity develops. Appetite maybe reduced, and this may contribute to decreased rate of gain. The clinical signs may be exacerbated by other respiratory infections (bacterial or viral). Atypical mild respiratory signs with low mortality resembling influenza have also been reported (Gottschalk and Taylor, 2006).
Less common manifestations may be seen in some outbreaks. Fatal septicemia may rarely be observed in neonatal pigs. In primary epidemics involving pregnant females, abortions (probably due to fever episodes) may be observed, especially when naïve sows/gilts from SPF herds are infected. Head tilt and drooping of one or both ears may be observed owing to middle ear infection associated with A. pleuropneumoniae infection.
Lesions
The gross pathological lesions are located mainly in the lungs and vary according to clinical course of disease. Pneumonia can be unilateral, bilateral, lobar, diffuse or multifocal. In acute cases there is consolidation that is dark red to black, interlobular edema, and mild to severe fibrinous pleuritis.
In peracute cases the trachea and bronchi are filled with a foamy, blood‑tinged mucus exudate, and there may be few other gross changes. In slightly later peracute cases, the pneumonic areas appear dark red purple and slightly to moderately firm yet resilient with little or no fibrinous pleurisy. On cut surface there is diffuse hemorrhage, and areas of necrosis are friable.
In acute cases, layers of fibrin are obvious on the pleural surface and infrequently on the epicardium and pericardium in animals that live for at least 24 hours after infection (Figure 9). The thoracic cavity usually contains a blood‑tinged fluid. Affected areas of the lung are firm, rubbery, and mottled dark red purple to lighter white in areas that contain abundant fibrin. On cut surface, the parenchyma is heterogeneous (Figure 10). There are areas of hemorrhage and other areas that are necrotic and friable. White lines composed of fibrin and white blood cells are observed surrounding necrotic areas and distending interlobular septa. In some areas, interlobular septa may be instead distended with hemorrhagic red fluid.
In chronic cases, fibrosis of the previously fibrinous pleuritis results in firm adhesions between visceral and parietal pleura. These areas often result in tearing of the lungs during removal at necropsy or in the slaughterhouse, leaving portions of the lung adhered to the thoracic wall (Figure 11). Resolution of non‐necrotic areasfrom acute infection results in remaining cavitated necrotic foci that are surrounded by scar tissue known as sequestra. In many less severe cases, lesions in the lung parenchyma resolve, leaving only fibrous pleural adhesions. It has been demonstrated that a high prevalence ofdorsocaudal pleuritis at slaughter is very suggestive of previous A. pleuropneumoniae pleuropneumonia (Gottschalk and Taylor, 2006).
7. Diagnosis
Confirmation of A. pleuropneumoniae pleuropneumonia and typing of strains
A. pleuropneumoniae pleuropneumonia should be suspected when typical clinical signs and gross lesions are observed. Differentials in peracute cases where lungs are dark red and edematous should include other diseases that may produce similar lesions such as classical swine fever, influenza, pseudorabies, septicemic salmonellosis, and erysipelas. In acute cases with typical fibrinohemorrhagic pleuropneumonia, A. suis and pleuritic strains of Pasteurella multocida should also be considered. Diagnosis is confirmed by culture, identification, and often typing of A. pleuropneumoniae. Lung samples for culture should come from lesioned areas of lungs collected from peracutely or acutely affected untreated animals. In chronic cases, where sequestra are in the lungs and/or fibrous pleuritis is observed at necropsy or in the slaughter house, A. pleuropneumoniae culture is usually negative. Surveillance of the herd using serology can be used to determine herd status. It is relatively easy to demonstrate A. pleuropneumoniae in pneumonic lesions from freshly dead animals. However, in some cases A. pleuropneumoniae can be overgrown by other bacteria such as P. multocida. Primary isolation of A. pleuropneumoniae from tissues and secretions may be carried out on 5% sheep blood agar with a cross‐streak of S. aureus or S. epidermidis. After aerobic incubation overnight (usually in the presence of 5% CO2), small colonies appear in the neighborhood of the streak (NAD requirement) surrounded by a clear zone of complete hemolysis. This allows a rapid presumptive bacteriologic diagnosis. For some serotypes (such as serotypes 7 and 12), the zone of hemolysis is usually less intense. Altered blood agars (“chocolate agar”) or PPLO agar supplemented with NAD or yeast extract allow the growth of the organism, but colonies are less distinctive on these media. Presumptive biochemical identification can be carried out by demonstrating the CAMP phenomenon and urease activity. Usually, molecular testing will help confirm the identity of A. pleuropneumoniae. Most isolates recovered from acutely infected animals are typical A. pleuropneumoniae, and its identification is relatively straightforward. However, when biochemically atypical isolates (for example, urease negative isolates), unexpected isolates recovered from the lungs without typical lesions, and/or untypable isolates are identified, the use of species‐specific PCR should be used to confirm the identity of the A. pleuropneumoniae. Biotype II (NAD‐independent) isolates have been recovered more frequently in recent years. These isolates might be misidentified as A. suis. In these cases, complete biochemical identification along with species‐specific PCRtests must be done before sending the isolate for serological serotyping. A. suis isolates can present strong cross‐reactions with rabbit sera against serotypes 3–6–8 of A. pleuropneumoniae, and without a correct identification, these isolates can be serotyped and reported as A. pleuropneumoniae.
Serotyping is still of major interest for A. pleuropneumoniae since different serotypes have different virulence potential. In addition, serotyping is essential for choosing the most appropriate bacterins when they are used in a preventive strategy. Only biochemically (typical) or genetically (atypical) confirmed isolates should be serotyped. Although serotyping using antibodies with a variety of classical serological tests, such as agglutination, coagglutination, ring precipitation, immunodiffusion, and indirect hemagglutination, can be used, there is a need for high titer and highly specific antisera. In addition, the presence of cross‐reactions is a problem with many serotypes, and the use of two to three types of tests is usually required to identify these serotypes. In fact, serotyping by the use of polyclonal antibodies can be seriously questioned. Indeed, some serotypes (such as serotypes 3, 6, 8,and 15) are highly difficult to differentiate. Using serological tests the prevalence of serotype 8 has been underestimated in both the United Kingdom and North America, since most strains had originally been typed as serotype 3 or 6 by serological methods. Serotypes 4 and 7 are also difficult to be differentiated using polyclonal antibodies. The use of monoclonal antibodies for serotyping has also been reported. These antibodies, especially those directed against the capsular epitopes, can differentiate, for example, serotype 1 from serotypes 9 and 11 and serotype 7 from serotype 4. However, monoclonal antibodies against the LPS will cross‐react between serotypes sharing common LPS epitopes. Some of these monoclonal antibodies even showed that LPS epitopes are common between A. pleuropneumoniae serotype 7 and Actinobacillus lignieresii. Serotypes 9 and 11 cannot be differentiated by serological tests (Gottschalk and Taylor, 2006).
To overcome the problems of serological serotyping, several molecular techniques (mostly PCR) have been developed to serotype A. pleuropneumoniae from pure cultures. For a recent review on the available techniques and their advantages/disadvantages. The most reliable PCR tests are based on cps locus genes; as it is the case with antibodies, it is important to note that serotypes 9 and 11 cannot yet be differentiated by the available PCR. Although PCR tests based on Apx toxin genes have been suggested to differentiate most serotypes, its use showed poor correlation when strains of known serotypes were tested. It is important to mention that using PCR tests (many of them based on cps locus genes) will not identify atypical strains with a combination of a given CPS serotype and a different LPS serotype as being described inNorth America, Europe, and Japan. Hence, there is still a need for reference laboratories using well‐standardized serological techniques to confirm the identity of such atypical strains. Since PCR tests can be performed by any laboratory with minimal infrastructure and without the need of serotype‐specific antisera, data on serotype distribution of isolates recovered from diseased animals in different countries will probably be available during the next years. Although not highly useful for serotyping, PCR toxin typing can be used to determine which Apx toxin genes are carried by a certain isolate, which may help to anticipate its virulence. For example, a non‐virulent serotype 1 strain recovered from healthy animals from a herd free of A. pleuropneumoniae ‐related disease was shown to produce ApxI only. Similarly, low virulent serotype 2 strains isolated in North America produces ApxII only, whereas highly virulent European strains produce both ApxII and ApxIII. Bacteriological diagnosis of chronic disease is more complex, because it is difficult to culture A. pleuropneumoniae from such lungs, especially when lesions are old. In the lungs that contain sequestra, direct detection of A. pleuropneumoniae antigens in lung tissues can be performed by extracting antigens and testing by ring precipitation, coagglutination, latex agglutination, ELISA, and/or counterimmunoelectrophoresis.However, results should be interpreted with caution, since most of these tests have not been validated in the field. Bacterial presence can also be observed in tissues by using either a fluorescent or an immunoperoxidase antibody test. However, these tests are serotype specific, and they will not detect all A. pleuropneumoniae strains. Nucleic acid from A. pleuropneumoniae (species specific) may also be detected by labeled DNA probes in tissue. Direct confirmation by PCR of the presence of A. pleuropneumoniae in lung tissue using A. pleuropneumoniae ‐specific PCR is not yet routinely used, although it has been used with success in a few cases (unpublished observations). When only fibrous pleuritic lesions are present, A. pleuropneumoniae antigen/DNA may not be detected in the lung. Therefore, serology along with disease history in the herd of origin may be used to determine herd status (Gottschalk and Taylor, 2006).
Detection of tonsillar carriers
The detection of A. pleuropneumoniae from clinically healthy carrier animals is even more complex. This may be required in cases of equivocal serological results of seed stock introduction into negative herds or in eradication programs. Bacteria are usually localized in tonsils and, less frequently, in the nasal cavities where they must be cultured in the presence of other commensal bacteria, including several other NAD‐dependent bacterial species. Selective media have been described, although their sensitivity is very low due to typical heavy contamination of plates. To overcome the presence of a highly contaminating flora, an immunomagnetic separation technique for the selective isolation of a given serotype of A. pleuropneumoniae from tonsils has been developed and applied to serotype 1 (in Canada) and serotype 2 (in Denmark). Additional field studies confirmed its better sensitivity when compared with the classical bacterial isolation method. Any specific serotype can be isolated using the magnetic beads by only changing the specificity of the antibody (unpublished data). Although this test presents good sensitivity, it is time consuming and expensive. On the other hand, it allows the isolation of live A. pleuropneumoniae for further use in antimicrobial testing or in autogenous vaccine production. It is important to mention that the species identity of any A. pleuropneumoniae‐like isolate recovered from a tonsil must be confirmed by PCR. Due to antigenic similarities, A. porcitonsillarum has already been isolated using magnetic beads coated with anti-serotype 1 immunoglobulins. Molecular techniques are presently used for the detection of A. pleuropneumoniae from tonsils. Several PCR techniques that amplify well‐defined sequences of the genome have been described for rapid and affordable detection of A. pleuropneumoniae, and ready‐to‐use PCR kits have been commercialized. PCR tests can be directly used with swine tonsil homogenates (direct PCR to detect live and killed bacteria) or after culture of tonsils (after‐culture PCR to detect only live bacteria). After‐culture PCR is considered to have a higher sensitivity. Although tonsil biopsies can be used, the sensitivity of this type of sample is lower than that of whole tonsils. Most PCR tests are specific for the species A. pleuropneumoniae and cannot differentiate among serotypes. Since most conventionalherds are infected with several low virulent serotypes, the significance of a positive result is difficult to interpret. To overcome this problem, serotype‐specific PCRtests have more recently been described; however, most of these tests have been validated with pure cultures (with a general goal of serotyping) rather than detection of carrier animals. Some of these serotype‐specific PCR with after‐culture methods have been successfully used. Finally, when detection of A. pleuropneumoniae is performed from tonsils collected at slaughter, results have to be interpreted with caution. Although (Fittipaldi et al., 2003) did not show any cross‐contamination at the slaughterhouse, another study suggested that cross-contamination principally by scald water during the slaughter process can occur. Finally, oral fluid has been shown to be a very low sensitive method to detect A. pleuropneumoniae after an experimental infection. An even much lower sensitivity is expected in samples from subclinically and chronically infected animals (Gottschalk and Taylor, 2006).
Serology
Serology is the preferred and most cost‐effective method for A. pleuropneumoniae surveillance. Serological testing has been used widely for the diagnosis, management, and eradication of virulent serotypes of A. pleuropneumoniae. In fact, serology is the most effective tool used to detect subclinical A. pleuropneumoniae infections. Some countries, such as Canada and Denmark, use serology for epidemiological surveillance of breeding herds on a routine basis. There are basically two types of serological tests: (1) those that detect all serotypes of A. pleuropneumoniae (without discrimination among serotypes) and (2) those that are serotype/serogroup specific. Commercial kits exist for both types of ELISA. There is only one test that can be considered A. pleuropneumoniae specific, since the antigen is produced by all A. pleuropneumoniae strains (with rare exceptions) and is not produced by any other bacterial species: the ApxIV ELISA test. However, few controlled and comprehensive studies regarding its use are available. It has been recently shown that the test presents an excellent specificity but, under the conditions tested, a relatively low sensitivity, since it correctly identified less than 13% of samples from A. pleuropneumoniae experimentally infected pigs, a conclusion also reached in a previous study. Interestingly, an increasing detection rate was observed when animals became older. Recently, a four‐plex fluorescent microbead‐based immunoassay was developed for the simultaneous detection of antibodies against ApxI, ApxII, ApxIII, and ApxIV. Using this method, and although false‐positive results probably due to cross‐reactions with antibodies against ApxI and ApxII were observed, a high sensitivity was obtained with the ApxIV antigen. Interestingly, many false‐negative sera previously tested by ELISA were positive when tested by this new highly sensitive test. Finally, interpretation of the ApxIV test must take into account that subclinically infected herds with animals harboring bacteria in tonsils only may not induce high levels of antibodies against A. pleuropneumoniae toxins. Moreover, some strains possess insertion sequences in their genome that abolish ApxIV production. Animals infected with such strains do not produce antibodies against this toxin (Gottschalk and Taylor, 2006).
Concerning the serotype/serogroup‐specific tests, the CFT has been largely used in Denmark and is still used in a few US laboratories; in fact, this test has been clearly shown to possess a very low sensitivity. Amazingly, some countries (such as China and Russia) still require a negative A. pleuropneumoniae CFT tests for imported swine even though the low sensitivity and propensity for false positives of the CFT are well known. The most commonly used test in thelast 20 years has been the ELISA, and among serotype/serogroup‐specific antigens, purified O‐chain LPS antigen (LPS‐ELISA) has been the most successful. A well‐characterized O‐chain LPS as a diagnostic antigen in a standardized ELISA for A. pleuropneumoniae was first reported in Canada in 1994. Serological tests for additional serotypes have subsequently been set up using similar antigens, individually or by mixing different serotypes. These ELISA tests may identify animals infected with the following serotypes/serogroups based on antigenic similarities of the O‐chain LPS: 1/9/11, 2, 3/6/8/15,4/7, 5, 10, 12, 13, and 14. Atypical biotype I North American A. pleuropneumoniae strains of serotype 13 present cross reactions with serotype 10 due to a similar LPS composition. In fact, when the diagnosis is directed toward specific serotypes/serogroups, the LPS- ELISA seems to be the test of choice, as previously reported. O‐chain LPS antigens are also used in blocking/inhibition ELISA tests using polyclonal or monoclonal antibodies. It has been shown that the LPS‐ELISA for serotype 1/9/11 showed low levels of cross‐reactions with animals experimentally infected with high doses of A. porcitonsillarum. In addition, animals infected with one atypical strain (without O‐chain LPS) did not induce antibodies detected by this ELISA. However, the latter two situations are exceptional and have been observed only once (unpublished data). As for the ApxIV test, multiplex fluorescent microbead based immunoassays for the identification of several serotypes (but identifying which ones are reacting) based on LPS and/or capsular antigens have been recently developed.
Tests detecting antibodies against specific serotype/serogroups and those detecting all serotypes of A. pleuropneumoniae are both useful, but they should be used appropriately. Although false‐positive results have been exceptionally described, high health status herds already known to be free of all serotypes of A. pleuropneumoniae may decide to use the ApxIV‐based test as a surveillance tool. It may be hypothesized that even though the ApxIV ELISA seems to present lower sensitivity than the LPS‐ELISA, an A. pleuropneumoniae strain that would have been introduced in a completely naïve herd would induce seroconversion and, depending on the strain, a relatively high seroprevalence. Under these circumstances, the ApxIV ELISA should significantly increase its sensitivity, although this remains to be demonstrated. If positive reactions are observed in herds, serological testing using antigens of different serotypes of A. pleuropneumoniae should be used. Otherwise, it would be extremely difficult to evaluate the level of risk of disease without knowing the serotype involved. In those cases, it is advisable to test all samples (positive and negative) with serotype/serogroup‐specific ELISA to confirm the diagnosis (Gottschalk and Taylor, 2006).
Most conventional herds are infected with one or more serotypes. It was shown that 70% of the Canadian herds tested had antibodies against A. pleuropneumoniae. In some herds, sows may be infected by more than one serotype, but only one of them is consistently transmitted to offspring and found in grower–finisher animals. The use ofthe ApxIV test would not be useful in these cases, since it is not able to evaluate the risk. As mentioned above, it is important for a country to have reliable updated data regarding the most important serotypes clinically affecting animals in order to determine which serotypes (orserogroups) should be tested serologically. Sera are still the main sample used for serology of A. pleuropneumoniae. LPS‐ELISA tests have been used experimentally with fluids collected from muscles of pigs as well as colostrum. Oral fluid samples have not been appropriately evaluated so far. Pooling sera from different individuals may significantly reduce the sensitivity of the test, especially in subclinically infected animals, and this practice is not recommended (unpublished observations). Even though serologic tests are valuable to identify subclinically infected herds and animals, serological testing occasionally generates ambiguous results. In such cases, molecular detection and/or bacterial isolation from tonsils could be carried out to clarify the herd status. In fact, tonsil colonization without induction of antibody response has been demonstrated. A. pleuropneumoniae is a very dynamic pathogen, and sometimes diagnosticians and practitioners must face atypical situations (Gottschalk and Taylor, 2006).
8. Treatment
Mark and separate the sick animal to avoid transmission and for better treatment
- Treatmet regimen A:
+ In the morning: Tiamulin 1ml/10kg bodyweight, intramuscular injection, once a day, in 3-5 days
+ In the eveening: Spectylo 1ml/10kg body weight, intramuscular injection, once a day, in 3-5 days
-Combined treatment:
+ Bcomplex fortified: 1ml/10kg bodyweight
+Vitamin K: 1ml/10kg bodyweight. Once a day, in 3-5 days consecutively
+Atropin: 1ml/5-10kg bodyweight. Once a day, in 3-5 days consecutively
+Vime- Electrolyte: 1g/2-4liters of water
Administrate orally during daytime as needed
-Treatment regimen B:
+ In the morning: Tylovet 1ml/10kg bodyweight, intramuscular injection, once a day, in 3-5 days
+In the evening : Vimetryl 5: 1ml/10kg bodyweight, intramuscular injection, once a day, in 3-5 days
-Combined treatment:
+ Bcomplex fortified: 1ml/10kg bodyweight
+ Vitamin K: 1ml/10kg bodyweight. Once a day, in 3-5 days consecutively
+ Atropin: 1ml/5-10kg bodyweight. Once a day, in 3-5 days consecutively
+ Vime-Electrolyte: 1g/2-4 liters of water.
Administrate orally during daytime as needed
9. Control and prevention of A. pleuropneumoniae
9.1 Control and prevention
When herds are free of A. pleuropneumoniae, strict biosecurity measures should be applied to prevent introduction of the organism. The greatest risk is presented by introduction of potentially infected (clinically healthy) pigs. Seedstock should be purchased from herds with a history of absence of clinical sign, lesions, and negative serological testing for A. pleuropneumoniae. Ideally, incoming animals should also be quarantined and serologically tested as negative before introduction. If the herd where the animals will be introduced is already positive for certain serotypes known to be of low virulence, similar precautions to introduction of serotypes known to be generally more virulent should be practiced. Introduction of animal carriers of low virulent strains of A. pleuropneumoniae in an SPF A. pleuropneumoniae‐negative herd should be avoided. Indeed, most strains recovered from clinical cases and belonging to serotypes of lower virulence in Canada (serotypes 6, 12, and 15) are usually isolated in high health status herds, which were previously free of all serotypes (unpublished observations). In herds that are already infected by virulent serotypes, A. pleuropneumoniae-negative seedstock should be vaccinated with products appropriate for the infecting virulent serotypes according to label directions, and time should allow for development of immunity before introduction. During outbreaks of pleuropneumonia on A. pleuropneumoniae infected farms, the first priority must be to control mortality by the treatment of affected individuals, usually including all contact animals in the affected pen. Treatment of animals in surrounding pens should be evaluated upon the extension of clinical disease; in fact, animals should be allowed to develop natural immunity. Generally, good environmental management minimizes outbreaks in infected herds and includes maintaining appropriate environmental temperature with minimal fluctuations, seasonally appropriate ventilation, use of solid partitions between pens, all‐in/all‐out movement of pigs, appropriate stocking densities, and younger weaning ages (<21days) (Gottschalk and Taylor, 2006).
The balance between disease resistance and tolerance will determine A. pleuropneumoniae load and host damage (Figure 12). However, this can be difficult to experimentally determine as: (1) the same cell types, e.g. macrophages, are involved in both disease resistance and tolerance; and (2) it often requires disease resistance to be stably maintained, e.g. through the use of antibiotics. One approach is to plot disease (health) parameters versus pathogen load over time, and the fitness curves can potentially be used to estimate variations in disease tolerance. While A. pleuropneumoniae load is relatively easy to quantify, choice of an informative health parameter to measure is considerably more difficult. Nevertheless, such disease curves have the potential to identify parts of the A. pleuropneumoniae disease process that are worthy of exploration. An alternative way of thinking, that reflects the overlap between disease tolerance and resistance, is to consider inflammation as the extreme end of a spectrum that ranges from hemostasis to the stress response to the inflammatory response. In this model, inflammation can be triggered either by: (1) extreme deviation of regulated variables (e.g. temperature) from normal values, and is classed as a stress response; or (2) a challenge (e.g. infections, toxins, or tissue damage) that can cause deviation of regulated variables, but is not itself a regulated variable, and is classed as a defense response. Thus, inflammation has both stress and defense response components.
Prevention regimen: Use antibiotics for other pigs in the herb which has sick pigs or recovering pigs
- Antibiotic: Tiamulin 1kg/1,5 tons of feeding food, use in 7 days consecutively
- Combined treatment:
+ Vime C Electrolyte: 1g/2-4litters of water. Administrate orally during daytime as needed.
+ Vitamin B complex: 1g/ 3 liters of water or 1kg/ 1-2 tons of feeding food.
Shed disinfection: To avoid other pathogens’s transmission and superiinfection.
- In infected shed: use antiseptic once a day in 3-5 days
- In non-infected shed: use antiseptic 1-2 times a week
+ Altacid: 1 liter/ 100liters of water, spray directly on cattle, spray on all surfaces of the shed (in and out) and the entrance.
+Vimekon: 1kg/200 liters of water, spray directly on cattle, spray on all surfaces of the shed (in and out) and the entrance.
9.2 Vaccination
A wide range of vaccines have been developed for this disease. These vaccines can basically reduce clinical signs and lung lesions, but they are poorly effective in eliminating the carrier stage. Commercial vaccines fall into two main groups: killed organisms (bacterins) and subunit toxin‐based vaccines. Lately, a combination of these types of vaccines (bacterins + toxins) appeared in the market. Vaccination with bacterins is serotype specific; possible cross-immunity with cross‐reacting serotypes has been suggested, although it still should be confirmed. The use of a bacterin should be done only in farms where the involved serotype has been identified, although animals vaccinated with bacterins will produce antibodies that might cross‐react with ELISA tests that use O‐LPS as antigens. However, this is not always the case: diagnostic tests have been developed to detect infected animals using highly purified antigens. Vaccination with bacterins may induce high levels of antibodies against a variety of surface antigens (including the CPS). In some cases, the level of antibodies against the O‐chain LPS in vaccinated animals may not be high enough to be detected in the ELISA test: this is not an indication of failure of the vaccine on its capacity to induce antibodies. Many commercial bacterins induce protection even if they do not induce such cross‐reacting antibodies detectable by the O‐chain ELISA kit. Finally, in some countries, autogenous vaccines are used: this approach may only be useful when serotypes involved in clinical cases are not included in available commercial bacterins. In the latter case, another option may also be the use of toxin‐based vaccines that would provide protection against all serotypes.
Subunit vaccines, composed of the purified three major RTX exotoxins (ApxI, ApxII, and ApxIII) with or without a 42kDa outer membrane protein of
A. pleuropneumoniae
, or in suspensions mixed with bacterins, have been developed and shown to give protection against all major serotypes under experimental conditions as well as in field trials. A potential protection conferred by the ApxIV toxin, produced by all strains in vivo, seems to be controversial, and it has not yet been incorporated in any commercial vaccine. Keeping in mind the very complex pathogenesis of porcine pleuropneumonia, inclusion of other bacterial virulence factors in vaccines might also be of value. A wide range of antigens administered by either parenteral, aerosol, or oral routes have been found to be experimentally protective, but none of them have been validated in the field. Finally, live vaccines using laboratory obtained non‐virulent mutants have also been developed and showed to protect against homologous and heterologous serotypes. Some of these live vaccines even use the differentiating infected from vaccinated animals (DIVA) concept. Vaccines may provide high levels of protection against morbidity in experiments, reduce mortality, reduce the number of treatments required, increase daily live weight gain, and improve feed conversion efficiency. The quality of the carcass is also improved, with fewer condemnations for pneumonia and lower slaughtering costs through reductions in pleurisy and pericarditis. The decision to vaccinate should be carefully evaluated; the costs of mortality alone should not be the sole consideration, because the other effects on productivity listed above contribute to the benefits of vaccination. In some cases, individual and intensified medical treatments of affected pigs may be needed to reduce the impact of A. pleuropneumoniae.
Vaccination of piglets is usually advised; animals should not receive the first dose during the first weeks of age to avoid interference with maternal antibodies. Sows can also be vaccinated without adverse effects, and replacement animals can be vaccinated before their introduction in an infected herd. Vaccination of sows with serotype specific bacterins may reduce/ delay colonization of piglets, which may lead to a reduction, under certain circumstances, of clinical signs in grower finisher pigs (unpublished observations). Since antibodies against somatic antigens are needed, this effect would not be possible with exclusively toxin‐based vaccines. Finally, the presence of antibodies (either natural or vaccine induced) will not eliminate the carrier state of animals at the tonsil level (Gottschalk and Taylor, 2006).
10. References
Auger, E., Deslandes, V., Ramjeet, M., Contreras, I., Nash, J.H., Harel, J., Gottschalk, M., Olivier, M., Jacques, M., 2009. Host-pathogen interactions of Actinobacillus pleuropneumoniae with porcine lung and tracheal epithelial cells. Infection and immunity 77, 1426-1441.
Bertram, T., 1988. Pathobiology of acute pulmonary lesions in swine infected with Haemophilus (Actinobacillus) pleuropneumoniae. The Canadian Veterinary Journal 29, 574.
Bertram, T., 1990. Actinobacillus pleuropneumoniae: molecular aspects of virulence and pulmonary injury. Canadian Journal of Veterinary Research 54, S53-56.
Casadevall, A., Pirofski, L.-a., 2018. What is a host? Attributes of individual susceptibility. Infection and immunity 86, e00636-00617.
Devenish, J., Rosendal, S., 1989. Identification of the heat-labile hemolysin of Actinobacillus pleuropneumoniae serotype 1. Canadian Journal of Veterinary Research 53, 251.
Dubreuil, J.D., Jacques, M., Mittal, K.R., Gottschalk, M., 2000. Actinobacillus pleuropneumoniae surface polysaccharides: their role in diagnosis and immunogenicity. Animal Health Research Reviews 1, 73-93.
Fittipaldi, N., Broes, A., Harel, J., Kobisch, M., Gottschalk, M., 2003. Evaluation and field validation of PCR tests for detection of Actinobacillus pleuropneumoniae in subclinically infected pigs. Journal of clinical microbiology 41, 5085-5093.
Frey, J., Nicolet, J., 1988. Purification and partial characterization of a hemolysin produced by Actinobacillus pleuropneumoniae type strain 4074. FEMS microbiology letters 55, 41-45.
Gottschalk, M., Taylor, D., 2006. Actinobacillus pleuropneumoniae. Diseases of swine 9, 563-576.
Kamp, E.M., Popma, J.K., Anakotta, J., Smits, M.A., 1991. Identification of hemolytic and cytotoxic proteins of Actinobacillus pleuropneumoniae by use of monoclonal antibodies. Infection and Immunity 59, 3079-3085.
Kristensen, C., Angen, Ø., Andreasen, M., Takai, H., Nielsen, J., Jorsal, S., 2004. Demonstration of airborne transmission of Actinobacillus pleuropneumoniae serotype 2 between simulated pig units located at close range. Veterinary microbiology 98, 243-249.
Liggett, A., Harrison, L., Farrell, R., 1987. Sequential study of lesion development in experimental haemophilus pleuropneumonia. Research in veterinary science 42, 204-212.
Nielsen, R., 1975. Colostral transfer of immunity to Haemophilus parahaemolyticus in pigs. Nordisk veterinaermedicin 27, 319-328.
Nielsen, R., Mandrup, M., 1977. Pleuropneumonia in swine caused by Haemophilus parahaemolyticus. A study of the epidemiology of the infection. Nordisk veterinaermedicin 29, 465-473.
Rosendal, S., Mitchell, W., Weber, M., Wilson, M., Zaman, M., 1980. Haemophilus pleuropneumonia: lung lesions induced by sonicated bacteria and sterile culture supernatant [swine], 1980 International Pig Veterinary Society Congress: Proceedings, Copenhagen (Denmark), 30 Jan-3 Jul 1980. IPVS.
Rycroft, A.N., Garside, L.H., 2000. Actinobacillus species and their role in animal disease. The Veterinary Journal 159, 18-36.
Wilson, B.A., Ho, M., 2013. Pasteurella multocida: from zoonosis to cellular microbiology. Clinical microbiology reviews 26, 631-655.
Zhuang, Q., Barfod, K., Wachmann, H., Mortensen, S., Lavritsen, D., Ydesen, B., Willeberg, P., 2007. Risk factors for Actinobacillus pleuropneumoniae serotype 2 infection in Danish genetic specific pathogen‐free pig herds. Veterinary record 160, 258-262.
Žutić, M., Ašanin, R., Milić, N., Ivetić, V., Vidić, B., Žutić, J., Ašanin, J., 2008. Isolation and identification of Actinobacillus pleuropneumoniae in pig's lungs at farms and their sensitivity to antibiotics. Acta Veterinaria-Beograd 58, 499-507.
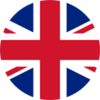