To My Quyen, Tran Duy Thanh, Pham Trang Thanh Nguyen,
Nguyen Khanh Thuan, Nguyen Phuc Khanh, Nguyen Thanh Lam*
1. Introduction
Swine dysentery (SD) is a severe mucohaemorhagic enteric disease of pigs, which has a large impact on pig production and causes important losses due to mortality and sub-optimal performance. This disease is caused by Brachyspira hyodysenteriae that has been traditionally considered a pathogen mainly transmitted by direct contact, through the introduction of subclinically infected animals into a previously uninfected herd, recent findings position B. hyodysenteriae as a potential threat for indirect transmission between farms (Alvarez-Ordóez et al., 2013). Besides that, this disease is most often observed in grower–finisher pigs, wherein susceptible pigs develop a significant mucohemorrhagic typhlocolitis following infection with strongly hemolytic spirochetes of the genus Brachyspira (Burrough, 2016). Specifically, SD primarily affects pigs during the growth and finishing periods, and clinical signs, which range from mild, mucous diarrhoea with unaltered general condition to severe haemorhagic diarrhoea with a mortality rate of 50–90% (Hampson et al., 2006), seem to occur in a cyclic manner at 3 to 4 weeks intervals, with these recurring symptoms often appearing only after removal of therapeutic antibiotics (Suh and Song, 2005).
2. Aetiology
2.1 Bacterial characteristics
Brachyspira (previously, Serpulina or Treponema) hyodysenteriae, a spirochete, is the etiologic agent. It has axial filaments, is Gram-negative, anaerobic and the most pathogenic strains are strongly β-hemolytic (Hampson and Burrough, 2019). Those agents inhabit the large intestines of many species including pigs, rodents, dogs, and birds. Infection with these bacteria results in varying levels of clinical disease ranging from an inapparent carrier state to severe bloody diarrhea, and this variance depends upon the species of animal infected and the particular species of Brachyspira involved. Co-infections with both highly virulent and avirulent Brachyspira spp. may also occur (Burrough, 2012).
Brachyspira spp. has three complete genomes deposited in NCBI, and its genome size consists of a ~3-Mb chromosome and a ~36-kb plasmid. This plasmid is conserved among several strains, but it is not found in any non-virulent isolated strain in the field, suggesting that it may be an essential virulence factor for the species (de Jesus Sousa et al., 2020).
Table 1. Characteristic features of the genus Brachyspira
Brachyspira | |
Morphology | 6-14 regular spirals with 1 µm amplitude |
Length | 5-20µm
|
Width | 0.1-0.5µm |
Periplasmic flagella | 6-10 |
Insertion discs | 1 |
Ends | Pointed |
Atmosphere | Microaerophilic or anaerobic |
Arthropod vectors required | No |
Direct microscopy: Dark - field DCF stain Giemsa Silver impregnation FA technique Movement |
+ + Poor + + Rotation and majestic translational movement and stiff flexion |
The Brachyspira species are anaerobic but can tolerate brief oxygen exposure. They grow slowly in vitro and can easily be overgrown by other anaerobic members of the enteric microbiota unless selective isolation medium is used. Agar should be made selective with the addition of 400μg/mL spectinomycin and 25μg/mL each of colistin and vancomycin to make CVS medium (Jenkinson and Wingar 1981). The more selective BJ medium (Kunkle and Kinyon, 1988) containing lower concentrations of the three antimicrobials, but with 25μg/mL spiramycin and 12.5μg/mL rifampin added, can also be used for agents of SD, but is not recommended for B. pilosicoli as it may inhibit growth. Typical Brachyspira form a low flat film of growth after 3–5 days at 98.6–108°F (37–42°C), without forming colonies. A zone of beta‐hemolysis surrounds growth on plates containing 5% defibrinated ovine or bovine blood. Hemolysis is strong for B. hyodysenteriae, B. hampsonii, and B. suanatina and weak for the other Brachyspira species. Atypical weakly hemolytic isolates of B. hyodysenteriae do occur. Strongly hemolytic isolates typically display a characteristic enhanced hemolysis, or ring phenomenon, around defects or slits in the agar (Figure 2).
2.2 Classification
Species of Brachyspira | Symptoms | Host |
B. hyodysenteriae, B. suanatina, and the novel B. hampsonii | Cause severe mucohemorrhagic diarrhea in pigs | Pigs |
B. innocens | Cause weakly beta-hemolytic | Pigs, chickens and/or humans |
B. pilosicoli, B. murdochii, B. intermedia, B. aalborgi, and B. alvinipulli | Cause diarrhea and/or colitis | Pigs, chickens and/or humans |
B. hyodysenteriae | Cause SD, which is a disease characterized by mucohemorrhagic diarrhea | Pigs |
Table 2. Additional information about genus Brachyspira (Mirajkar et al., 2016)
The genus Brachyspira contains nine officially recognized and several unofficial species. Seven Brachyspira species colonize swine: the three most common pathogenic species are B. hyodysenteriae and B. hampsonii, both agents of SD, and B. pilosicoli, the cause of PIS (or PCS). Of the other potentially pathogenic species, B. suanatina is an agent of SD that has only been reported in Scandinavia (Mushtaq et al.,2015), and some strains of B. murdochii and B.intermedia may occasionally cause colitis in swine. The final species colonizing swine is B. innocens, a nonpathogenic commensal (Hampson and Burrough, 2019).
Figure 3: Relationships between the nine officially named Brachyspira species based on the 16S rRNA gene sequences of the type strains. The seven species that are known to colonize pigs are marked in bold (Hampson and Burrough, 2019).
Useful features that may help to distinguish between Brachyspira species include their strength of beta‐hemolysis, their ability to produce indole, and their enzymatic profile in the commercial API‐ZYM kit (Fellströmet al. 1997). Some comparative features of the porcine spirochetes are presented in Table 3.
Table 3. Differentiation of seven Brachyspira species that colonize swine using strength of hemolysis on Trypticase soy blood agar, biochemical reactions, and utilization of sugars (Hampson and Burrough, 2019).
Species | Hemolysis | Indole | Hippurate | API-ZYMa |
B. hyodysenteriae | Strongb | +c | − | 1 |
B. hampsonii | Strong | − | − | 3 |
B. suanatina | Strong | +d | − | 5 |
B. intermedia | Weak | + | − | 1 |
B. innocens | Weak | − | − | 2 |
B. murdochii | Weak | − | − | 3 |
B. pilosicoli | Weak | −c | +e | 4 |
a Reactions in the commercial API‐ZYM test:
1) Alpha‐glucosidase positive; alpha‐galactosidase negative.
2) Alpha‐glucosidase positive or negative; alpha‐galactosidase positive.
3) Alpha‐glucosidase negative; alpha‐galactosidase negative.
4) Variable reactions, including positive reactions for both enzymes; beta‐glucosidase negative.
5) Alpha‐galactosidase negative; beta‐glucosidase positive.
b Weakly hemolytic strains of B. hyodysenteriae have been recorded.
c Indole‐negative strains of B. hyodysenteriae and indole‐positive strains of B. pilosicoli have been recorded.
d Weakly positive indole.
e Hippurate‐negative strains of B. pilosicoli have been recorded.
+ positive reaction; − negative reaction.
2.3. Resistance
B. hyodysenteriae is relatively resistant in moist feces. It survives in feces diluted in water for 48 days at temperatures from 32 to 50°F (0–10°C), for 7 days at 77°F (25°C), and less than 24 hours at 98.6°F (37°C). In another study it survived for 10 days in soil at 50°F (10°C), for 78 days in soil in the presence of 10% pig feces, and for 112 days in pure pig feces. Drying of dysenteric feces rapidly kills B. hyodysenteriae. Phenolics and sodium hypochlorite are the most effective disinfectants (Hampson and Burrough, 2019).
There are a few avirulent or weakly beta-hemolytic Brachyspira strains that are predominantly nonpathogenic. Other organisms in the large intestine, especially anaerobes, may facilitate colonization and lesion formation. B. hyodysenteriae has the ability to survive under a wide range of environmental conditions but is susceptible to heat, ultraviolet (UV) light, and desiccation, as well as soaps and disinfectants (Iastate.edu).
The Brachyspira species are anaerobic but can tolerate brief oxygen exposure. They grow slowly in vitro and can easily be overgrown by other anaerobic members of the enteric microbiota unless selective isolation medium is used. Agar should be made selective with the addition of 400μg/mL spectinomycin and 25μg/mL each of colistin and vancomycin to make CVS medium (Hampson and Burrough, 2019).
3. Pathogenicity
B. hyodysenteriae was shown to differ from all the other spirochaetes, including Leptospira, Borrelia and Treponema, in signal transduction and in amino acid transport and metabolism systems. A relative paucity of signal transduction mechanisms relative to the genome size, which probably reflects the relatively narrow ecological niche occupied by the microorganism in the porcine large intestine, was observed. On the other hand, the proportion of genes involved in amino acid transport and metabolism was relatively high, and this probably reflects the adaptation to the environment of the intestinal tract, where proteins from host cells and dietary ingredients are abundant. It was also noteworthy the high proportion of putative protein-coding sequences (CDS) showing high similarity to proteins from the genus Escherichia and Clostridium. It is likely that these genes were involved in horizontal gene transfer events involving B. hyodysenteriae and one or more Clostridium and Escherichia species. Since they inhabit the same environment in the large intestine, opportunities for gene exchange favouring their survival in this niche are abundant. Several CDS predicted as putative virulence factors were identified. These included proteases involved in virulence via the destruction of host tissues, and ankyrin proteins, known to bind to the host chromatin playing a critical role in the interaction with the host cells. Moreover, seven potential hemolysin production genes, ten flagella-associated genes that can form part of a type III secretory system, at least 84 putative genes associated with chemotaxis and motility, and the key genes necessary for lipooligosaccharide biosynthesis were identified and proposed as virulence factors. Other authors have also identified various virulence life-style factors (e.g., outer membrane proteins, NADH oxidase, proteins of iron metabolism) with a predicted role in B. hyodysenteriae pathogenicity. The presence of a 35,940 bp circular plasmid in B. hyodysenteriae strain WA1 was also confirmed by Bellgard and co-workers. Interestingly, a recent study by La and colleagues has found evidence that this plasmid contributes to B. hyodysenteriae virulence. These authors have shown that the WA1 plasmid contains genes encoding enzymes forming part of the rhamnose biosynthesis pathway (rfb genes) that are predicted to function in incorporation of rhamnose in the O-antigen backbone of the cell wall lipooligosaccharide. In addition, other glycosyltransferases were shown to be encoded by the plasmid, and these may be involved in incorporating other sugars into the lipooligosaccharide (Alvarez-Ordóez et al., 2013).
4. Epidemiology
4.1 Susceptible hosts
Agents of SD naturally infect pigs (including feral pigs) and some species of birds (rheas, chickens, ducks, and geese). On infected farms, different agents of SD have been isolated from mice, rats, dogs, and waterfowl. Waterfowl appear to be a significant reservoir and potential means of introduction and spread for B. hampsonii, as this agent has been recovered from migratory waterfowl samples in North America and Europe and an avian origin isolate of B. hampsonii induced SD when experimentally inoculated into. Similarly, B. suanatina has been recovered from waterfowl in Sweden and Denmark, and an avian‐origin isolate induced clinical signs of SD following experimental inoculation in pigs (Hampson and Burrough, 2019).
4.2 Transmission
On endemically infected swine farms, transmission mainly occurs by ingestion of feces containing spirochetes. This is especially likely in single‐site, farrow‐to‐finish herds with continuous flow and poor biosecurity. Agents of SD may be spread by contact with clothing or footwear of animal caretakers that are contaminated with pig feces. Transmission between pens may occur in housing systems where there are open channels between pens. Lagoon water containing effluent can be a source of infection and should not be recycled. Feral pigs, other animals including rats and mice, and waterfowl that access farms or their water supplies are potential reservoirs that may transmit infection (Hampson and Burrough, 2019).
Outbreaks also occur in herds following transmission via rodents or birds from adjacent infected farms, by introduction through contaminated feed or animal trucks, or by visitors who have had contact with infected pigs. Robertson et al., found that allowing visitors onto farms and the presence of rodents were both associated with disease. In contrast, heightened biosecurity, such as the provision of boots and protective clothing for visitors, the presence of security fencing, on‐site feed mixing, and obtaining replacement breeders from a consistent source, were protective. B. hyodysenteriae is shed in the feces for variable periods of time and recovered asymptomatic pigs may transmit infection to susceptible pigs for at least 70 days (Hampson and Burrough, 2019).
Moreover, transmission of B. hyodysenteriae is by ingestion of infectious feces. It can persist in lagoon water for at least two months, moist feces for two months, and soil for 18 days. Mice have been reported to shed B. hyodysenteriae for 180 days and dogs for 13 days. The organism can be transmitted by birds, flies, and fomites. Carrier swine can transmit the agent for at least 90 days. Clinically normal, purchased carriers, including breeding stock, often are the source of initial exposure. Carrier sows often transmit to their piglets. Infected mice on premises may also be a source of infection (Iastate.edu).
4.3 Environment factors
Although SD is a multifactorial disease which pathogenesis is complex and poorly understood, several factors have been associated with the occurrence of the condition. Thus, the outcome of infection by B. hyodysenteriae might be influenced by age (Olson, 1974), stress, acid secretion in the stomach (Savage, 1980), differences in the dose of the infectious agent, diet and the virulence of B. hyodysenteriae strains (Hampson et al., 1992). The following sections of the review emphasize the main environmental factors determining disease establishment and transmission, i.e., environmental survival of the pathogen, husbandry factors, role of vectors, relevance of pig’s diet, and interaction of the microorganism with gut microbiota (Hampson and Burrough, 2019).
The determinant factor of the disease is the pathogen B. hyodisenteriae whose persistence in the environment is enhanced by low temperatures and the presence of faeces. Factors which promote the transmission, establishment and persistence of the disease are the presence of vectors, diets with high energy and proteins, and bad husbandry practices; the role of highly fermentable diets is unclear. In contrast, all-in/all-out (AI/AO) management together with cleaning and disinfection, strict biosecurity and highly digestible diets are preventive factors to the establishment of SD. Finally, the production system is a decisive factor to a great extent in the control and prevention of the disease. Farrow-to-finish herds have lower risk of SD entrance but in contrast the persistence of the pathogen is higher than in multi-site production systems (Alvarez-Ordóez et al., 2013).
5. Pathogenesis:
B. hyodysenteriae is ingested with clinical signs developing 5-21 days later; incubation period is dependent upon dose but the infective dose is quite small (Iastate.edu).
The pathogenesis of SD is incompletely understood and has been recently reviewed. Individual animal susceptibility to development of SD varies and is, in part, due to differences in the colonic microbiota. Gnotobiotic pigs are variably colonized by B. hyodysenteriae but do not develop SD, while gnotobiotes inoculated with intestinal scrapings or content from pigs with dysentery readily develop disease. These findings suggest that infection with other microorganisms along with an agent of SD is required for disease expression. Anaerobes such as Bacteroides vulgatus and Fusobacterium necrophorum were shown to successfully fulfill this requirement.
Following ingestion, B. hyodysenteriae survives the acidic environment of the stomach and eventually reaches the large intestine where they colonize and produce disease. Experimentally, an inoculum of 105–1010 colony‐forming units (cfu) may produce SD (Kinyon et al., 1977). Proliferation and colonic mucosal colonization are aided by the ability of B. hyodysenteriae to utilize available substrate, penetrate and move through viscous mucus down a chemotactic gradient into the crypts, and avoid potential oxygen toxicity at the surface of the colonic mucosa. Clinical signs and lesions start to develop as bacterial cell numbers reach 106/cm2 of mucosa. Spirochetes typically appear in the feces 1–4 days before onset of SD, concurrent with a shift in colonic bacteria from predominantly gram‐positive to mainly gram-negative species; however, preclinical shedding of up to 8 days has been reported (Hampson and Burrough, 2019).
The mechanisms of tissue destruction in SD are not fully understood. There is a loss of sulfated mucins in the colonic mucosa of pigs with acute SD relative to controls, and the mucus layer becomes disorganized and lacks the striations apparent in age‐matched control pigs. Additionally, mucin production increases dramatically in pigs with SD due to the de novo production of mucin 5AC and increased production of mucin 2. Increased mucus affords increased binding sites for spirochetes, and alteration of the barrier structure may allow improved access to the underlying epithelium. The hemolysin(s) and LOS may then cause local damage, resulting in epithelial sloughing. Subsequent mucosal and submucosal invasion by secondary bacteria and the protozoan Balantidium coli may contribute to lesion formation.
Diarrhea appears to result from failure of epithelial transport of sodium and chloride ions from lumen to blood resulting in colonic malabsorption, and not from the activity of prostaglandins released from the inflamed mucosa or spirochetal enterotoxins, or from direct tissue damage induced by spirochetal cell components. Occasional peracute deaths may arise from endotoxin release (Hampson and Burrough, 2019).
6. Clinical signs and pathology
6.1 Clinical signs
SD occurs mainly in grower and finisher pigs and less frequently in weaner pigs. It may begin a few weeks after animals are moved from the nursery, coinciding with a dietary change and removal of antimicrobials used to control respiratory and enteric diseases. Older suckling piglets from gilts that have not been exposed to B. hyodysenteriae and piglets in newly infected herds are occasionally affected.
The first evidence of SD is usually soft, yellow to gray feces. Partial anorexia and increased rectal temperature of 104–105°F (40–40.5°C) may occur; however, a febrile response is not a consistent feature or predictive of spirochete shedding (Jacobson et al., 2007). A few hours to days after onset of clinical disease, large amounts of mucus and often flecks of blood are found in the feces. This progresses to watery feces containing blood, mucus, and shreds of white mucofibrinous exudate (dysentery) with concurrent staining of the perineum. Most pigs recover over several weeks, but their growth rate remains depressed. Prolonged diarrhea leads to dehydration, and the animals become weak and emaciated. The incubation period for SD is variable, ranging from 4 days to 3 months, but disease usually occurs within 10–14 days in naturally exposed pigs. Disease usually spreads gradually, with new animals becoming affected daily. The course varies between individual animals. Occasional pigs are peracutely
affected and die within a few hours to days (Hampson and Burrough, 2019).
Figure 6. (A) Affected pigs often exhibit loose feces giving a “dribbling” appearance under the rectum (B) A range of fecal appearances in an SD infection (Schwartz, 2013).
Figure 7. A. Diarrhea with blood and mucus from a pig infected with B. hyodysenteriae (B). Spiral colon from a pig infected with B. hyodysenteriae revealing hemorrhage and abundant mucus along the mucosal surface (Burrough, 2012).
6.2 Pathology
Lesions are limited to all or part of the cecum through the descending colon with spiral colon being most consistently affected in acute SD. Distribution of lesions tends to be more diffuse in the later stages of the disease. Typical lesions in acute SD include hyperemia and edema of the large intestinal wall, mesentery, and mesenteric lymph nodes. The large intestinal mucosa is usually edematous with loss of the typical rugose appearance and is covered by mucus, fibrin, and flecks of blood (Figure 7). Contents are soft to watery and contain exudate.
As disease progresses, large intestinal mural edema decreases and mucosal lesions become more severe. Exudation of fibrin increases, forming thick pseudomembranes composed of fibrin, mucus, and blood. As lesions become chronic, mucosal exudates contain less mucus and blood and are typically composed of a thin layer of fibrinonecrotic exudate resembling lesions of enteric salmonellosis. Lesions can also be observed in the colons of clinically healthy pigs as segmental reddened areas of the mucosa usually covered with mucus, but with normal contents. Microscopic lesions are limited to the cecum, colon, and rectum. In the acute phase of disease, spirochetes are most numerous in the lumen and within superficial crypts (Figure 8) but may also be observed attached to luminal epithelium within disrupted epithelial and goblet cells, and in the superficial lamina propria, often in higher numbers surrounding blood vessels. The mucosa and submucosa are thickened due to vascular congestion, serofibrinous effusion, and infiltrating leukocytes, predominantly neutrophils. Erosion of luminal epithelium results from loss of cohesion, necrosis, and shedding of enterocytes. Superficial lamina propria subjacent to erosions may have neutrophilic cuffing of blood vessels, hemorrhages, and invasion by luminal bacteria and protozoa. Expulsion of mucus from goblet cells fills colonic crypts 3 days after onset of clinical disease, and hyperplasia of goblet cells occurs after 5 days (Jacobson et al. 2007).
Later changes include accumulation of fibrin, mucus, and cellular debris in mucosal crypts and on the luminal surface of the large intestine. Superficial necrosis of the mucosa may be extensive, but deep ulceration is not typical. Numbers of neutrophils, lymphocytes, and plasma cells increase in the lamina propria. Chronic mucosal lesions usually include less hyperemia and edema, more extensive superficial necrosis, and extensive superficial mucosal fibrinonecrotic exudate.
Figure 8. Spiral colon from a pig 10 days after intragastric inoculation with B. hampsonii. There is serosal hyperemia and mesocolonic edema. A segment has been opened to reveal mucosal thickening, hemorrhage, and watery contents containing mucus, fibrin, and blood. Source: Courtesy of Dr. Eric Burrough.
Figure 9. B. hyodysenteriae within a colonic crypt and adjacent epithelium of a pig with SD (Warthin–Starry; bar = 20μm). Source: Courtesy of Dr. Eric Burrough.
Figure 10. Representative histopathology sections from explants inoculated with the pathogenic (A, Warthin-Faulkner, & B, H&E), non-pathogenic B. hampsonii strain (C, H&E), negative control (D, H&E) and positive control (E, H&E) (Costa and Harding, 2020).
7. Diagnosis
Diagnosis in a large breeding and/or grower-finisher unit will be based upon on the history of how the disease has spread and for how long, the clinical signs, post-mortem examinations, laboratory tests on faecal smears and the isolation and identification of B. hyodysenteriae by serological or biochemical tests and DNA analysis. In a large unit it is essential that the disease is quickly distinguished from colitis caused by other organisms or dietary factors, due to the sheer numbers of pigs involved.
- Microbial culture: Selective culture for Brachyspira spp from clinical samples provides a high degree of diagnostic sensitivity for SD; however, the ability to recover spirochetes from clinical samples depends largely upon sample handling and the sample itself.
- Polymerase chain reaction (PCR): For many infectious agents, direct PCR from clinical samples is common and highly effective; however, it should be noted that for Brachyspira spp, direct PCR on clinical specimens may lack sensitivity relative to microbial culture.
- Enzyme-linked immunosorbent assay (ELISA)-based techniques for the detection of antibodies to lipopolysaccharide antigen are effective in differentiating B. hyodysenteriae infection from B. innocens; however, these assays are serotype specific and therefore not universally applicable.
Several diarrheal diseases of swine must be differentiated from SD. These include proliferative enteritis, whipworm infestation, gastric ulcers, spirochaetal colitis (B. pilosicoli), and salmonellosis. Severe whipworm infections mimic mucohemorrhagic SD and worms are not grossly visible until three to four weeks after infestation. Salmonellosis lesions usually are not confined to the large intestine, as are SD lesions. Lesions of salmonellosis tend to extend deeper into the mucosa as ulcers and may be patchy in distribution with less mucus. Necropsy of several pigs and microscopic study of the colon often are helpful in separating the two diseases. Dual infections can occur. Spirochaetal colitis is best diagnosed by necropsy of acutely affected pigs, histopathology, isolation of the weakly beta-hemolytic causative organism, and definitive identification biochemically or by PCR (Iastate.edu).
8. Immunity
Changes occur in antibody levels and in cell‐mediated reactivity in pigs with SD, but their importance is unclear. Serum IgG levels to B. hyodysenteriae correlate with duration of clinical signs, while IgA levels in the colon reflect recent exposure). Neither is strongly correlated with protection from SD.
Pigs recovered from SD may be protected against sub- sequent challenge with B. hyodysenteriae for up to 17weeks, although some animals (7–43%) remain susceptible and approximately 10% may only become protected after two bouts of disease. Immunity is partially serotype specific and directed against LOS antigens.
Complement components in conjunction with immune serum may be involved in clearance of B. hyodysenteriae from the colon. Cell‐mediated immunity also may be involved in protection as inhibition of peripheral blood leukocyte migration, a delayed hypersensitivity response, and a T‐cell proliferative response to B. hyodysenteriae antigens are found in convalescent pigs (Jenkins et al. 1982). CD8αα cell proliferation has been recorded in pigs recovered from SD. By comparing circulating leukocytes and lymphocyte subpopulations in pigs, Jonasson et al. (2004) deduced that γδ T cells and CD8+ cells may be associated with susceptibility to infection, while monocytes and CD4+ CD8+ T cells appear to be the major responding lymphocytes. Increased numbers of neutrophils, γδ T cells, and specific antibodies have been found during the recovery phase.
Following experimental infection or vaccination with a bacterin vaccine, there is some protection against serotypes of B. hyodysenteriae other than those to which the animals have been exposed. It is not known whether infection with one Brachyspira species confers any protection against infection with another species (Hampson and Burrough, 2019).
9. Treatment
- Treatment regimen A
- Antibiotics:
+ In the morning: Tiamulin:1ml/10kg bodyweight ( Intramuscular injection, once a day, in 3-5 days)
+ In the evening: Spectylo: 1ml/10kg bodyweight ( Intramuscular injection, once a day, in 3-5 days)
- Combined treatment: Administrate orally during daytime as needed
+ Bcomplex fortified: 1ml/10lg bodyweight
+Vitamin K: 1ml/10kg bodyweight (Once a day: in 3-5 days consecutively)
+Atropin: 1ml/5-10kg bodyweight ( Once a day: in 3-5 days consecutively)
+Vime- Electrolyte: 1g/2-4 liters of water
- Treatment regiment B:
- In the morning: Tylovet 1ml/10kg bodyweight (Intramuscular injection, once a day, in 3-5 days)
- In the evening: Vimetryl 5: 1ml/10kg bodyweight (Intramuscular injection, once a day, in 3-5 days)
- Combined treatment: Administrate orally during daytime as needed
+ Bcomplex fortified: 1ml/10lg bodyweight
+Vitamin K: 1ml/10kg bodyweight (Once a day: in 3-5 days consecutively)
+Atropin: 1ml/5-10kg bodyweight ( Once a day: in 3-5 days consecutively)
+Vime- Electrolyte: 1g/2-4 liters of water
10. Control and prevention of swine dysentery
10.1 Control and prevention
Preventing the introduction of B. hyodysenteriae to negative herds is a high priority. Additions to the herd should be only from herds known to be free of swine dysentery. They should be added only after a quarantine period of 30-60 days. Some veterinarians recommend treating high-risk animals with tiamulin or carbadox during quarantine to reduce the possibility of carriers being introduced.
Three methods are used to eliminate swine dysentery and have been quite successful in the US:
- Early weaned piglets (<3 weeks) taken to a clean site and raised there usually remain free of B. hyodysenteriae.
- Meanwhile, the infected herd can be marketed, and the facilities cleaned and disinfected. This method allows one to retain valuable genetic stock.
- Segregated rearing with all in/all out production techniques has been quite successful in eliminating SD.
Medication is a second method: reduction of a herd to a minimal number of animals, followed by intensive treatment of those retained, is often used. Debilitated animals must be disposed of since they may not consume enough medicated feed or water. Prolonged, intensive treatment is expensive so numbers of swine to be treated should be minimized. Three widely used therapeutic agents include carbadox, lincomycin and tiamulin. Directions for their use should be followed carefully and every animal must be treated.
The third method of complete depopulation may be useful on premises with endemic swine dysentery where biosecurity and sanitation are difficult to implement. Depopulation should be done during warm, dry weather. During depopulation all facilities and equipment should be thoroughly cleaned and disinfected. The premises should be left vacant for at least two weeks, depending on the weather and level of achievable sanitation. B. hyodysenteriae usually will not survive for more than two weeks in soil or grass pens kept dry and relatively free of feces during dry, warm weather. Repopulation should be made with swine free of SD.
Any effort to eradicate SD requires an initial accurate diagnosis of SD, a commitment from the owners, and initial agreement on a protocol for accomplishing eradication and ongoing veterinary supervision. The eradication effort should be made during the warmest time of the year. A professionally-managed rodent control program is essential. Eradication must be coordinated with pig flow so that thorough cleaning and disinfection can be accomplished when the premises are vacant or the population minimal. If infected animals must be retained during the effort, a barrier system must be set up to separate clean from potentially contaminated areas.
10.2 Dietary interventions
The pigs’ diet is one of the most important factors regulating the microbial balance in the large intestine and can have a strong influence on colonisation by B. hyodysenteriae and on the occurrence or severity of clinical signs of SD. This suggests that control of SD may be achieved by manipulations of dietary ingredients or the use of specific dietary additives. Thus, Siba and co-workers demonstrated that pigs fed a highly digestible diet based on cooked white rice and animal protein were fully protected against SD, while pigs fed diets containing lupins and/or wheat displayed clinical signs of SD. Similar results have been obtained with other diets with low contents in soluble non-starch polysaccharides (sNSP) and resistant starch (RS), supporting the idea that microbial digestion of fermentable carbohydrates in the large intestine facilitates the occurrence of SD. However, protection against SD was also shown to be achieved through supplementation with highly fermentable carbohydrates (prebiotic diets).
Prebiotics are non-digestible food components which evade digestion by mammalian enzymes, in the upper regions of the gastrointestinal tract, reach the colon in an intact state and are then metabolized/fermented by beneficial members of the indigenous microbiota. Selective fermentation by such microorganisms may result in a healthier composition of the gut microflora and a lower susceptibility to gastrointestinal infections. Addition of prebiotics to the diet would therefore allow the manipulation of intestinal microbiota with the final aim of improving health and well-being and preventing SD. Accordingly, a high dietary concentration of inulin, whose possibly mechanisms of action have been mentioned above, has been reported to reduce the incidence of SD in pigs experimentally challenged with B. hyodysenteriae. Nonetheless, the reduction of the risk of developing SD was only achieved with a diet supplemented with high levels of inulin (80 g/kg) but not with lower levels, which is an expensive meal formulation. Finally, another dietary additive which has been reported to ameliorate SD, through immunomodulation and reduction of colonic inflammation, is conjugated linoleic acid (Alvarez-Ordóez et al., 2013).
10.3 Vaccination
Large efforts have been made in order to develop vaccines to control SD since Joens and co-authors reported that pigs which have recovered from acute SD are protected from disease when subsequently re-exposed to B. hyodysenteriae, indicating that the infection can induce a protective immune response. However, attempts to date have met with limited success. Tested vaccines have included whole-cell bacterins and orally administered attenuated strains. Bacterin vaccines may provide some level of protection but they do not provide adequate cross-protective immunity against strains of different serogroups, which would require the use of autogenous or multivalent bacterins. In addition, they are relatively expensive and difficult to produce on a large scale due to the fastidious growth requirements of the spirochaete. On the other hand, attenuated or genetically modified live avirulent vaccines may show reduced colonisation and cause less immune stimulation. An alternative approach may be to generate subunit vaccines that might be delivered by the expression of recombinant B. hyodysenteriae proteins on a bacterial delivery vector. Investigations into potential targets for such recombinant vaccines have focused on outer membrane proteins, flagelar proteins or iron storage proteins, although in most occasions recombinant vaccines tested have failed to provide enough protection in pigs (Alvarez-Ordóez et al., 2013).
10.4 Prevention regimen:
Antibiotic
Tiamulin: 1kg/1,5 tons of feeding food ( use in 7 days consecutively)
-Combined treatment:
+Vime C Electrolyte: 1g/2-4 liters of water ( Administrate orally during daytime as needed)
+ Vitamin B-complex: 1g/3 liters of water or 1kg/1-2tons of feeding food
11. References
Alvarez-Ordóez, A., Martínez-Lobo, F.J., Arguello, H., Carvajal, A., Rubio, P., 2013. Swine Dysentery: Aetiology, Pathogenicity, Determinants of Transmission and the Fight against the Disease. International Journal of Environmental Research and Public Health 10, 1927-1947.
Burrough, E., 2012. Brachyspira-associated colitis in swine, pig health, Professional Pig Community.
Burrough, E.R., 2016. Swine Dysentery: Etiopathogenesis and Diagnosis of a Reemerging Disease. Veterinary Pathology 54, 22-31.
CABI, 2021. Swine Dysentery. Invasive Species Compendium, Wallingford, UK: CAB International.
Costa, M.O., Harding, J.C.S., 2020. Swine dysentery disease mechanism: Brachyspira hampsonii impairs the colonic immune and epithelial repair responses to induce lesions. Microbial Pathogenesis 148, 104470.
de Jesus Sousa, T., Jaiswal, A.K., Hurtado, R.E., de Oliveira Tosta, S.F., de Castro Soares, S., Gomide, A.C.P., Alcantara, L.C.J., Barh, D., Azevedo, V., Tiwari, S., 2020. Chapter 5 - Pan-genomics of veterinary pathogens and its applications, in: Barh, D., Soares, S., Tiwari, S., Azevedo, V. (Eds.), Pan-genomics: Applications, Challenges, and Future Prospects. Academic Press, pp. 101-119.
Hampson, D., Cutler, R., Lee, B., 1992. Virulent Serpulina hyodysenteriae from a pig in a herd free of clinical swine dysentery. Veterinary Record 131, 318-319.
Hampson, D.J., Burrough, E.R., 2019. Swine dysentery and Brachyspiral colitis. Diseases of swine, 951-970.
Iastate.edu, Swine Dysentery and Spirochaetal Colitis, Swine Dysentery, Department of Veterinary Diagnostic & Production Animal Medicine.
Mirajkar, N.S., Phillips, N.D., La, T., Hampson, D.J., Gebhart, C.J., Fenwick, B.W., 2016. Characterization and Recognition of Brachyspira hampsonii sp. nov., a Novel Intestinal Spirochete That Is Pathogenic to Pigs. Journal of Clinical Microbiology 54, 2942-2949.
Olson, L., 1974. Clinical and pathological observations on the experimental passage of swine dysentery. Canadian Journal of Comparative Medicine 38, 7.
Schwartz, 2013. Bloody Scours (Swine Dysentery), A costly re-emerging disease that is preventable, Swine Health.
Suh, D.K., Song, J.C., 2005. Simultaneous detection of Lawsonia intracellularis, Brachyspira hyodysenteriae and Salmonella spp. in swine intestinal specimens by multiplex polymerase chain reaction. Journal of veterinary science 6, 231-237.
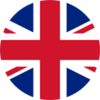